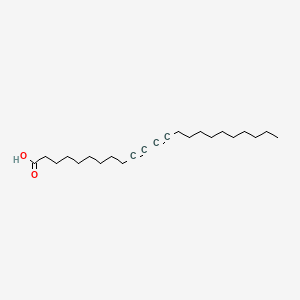
10,12-Tricosadiynoic acid
概要
説明
10,12-Tricosadiynoic acid (TRIC Acid), a conjugated diynoic fatty acid (C₂₃H₃₈O₂, molecular weight 346.55, CAS 66990-30-5), is a high-specificity, orally active inhibitor of peroxisomal acyl-coenzyme A oxidase 1 (ACOX1) . ACOX1 catalyzes the first step of very long-chain fatty acid β-oxidation, and its dysregulation is linked to hepatic lipotoxicity, oxidative stress, and non-alcoholic fatty liver disease (NAFLD) progression .
TRIC Acid functions as a prodrug: upon cellular uptake, it is converted to its active CoA ester form by peroxisomal very long-chain acyl-CoA synthetase (VLACS), irreversibly inhibiting ACOX1 with kinetic parameters KI = 680 nM and kinact = 3.18 min⁻¹ . Preclinical studies demonstrate that TRIC Acid (100 μg/kg orally in rats) reduces hepatic lipid accumulation, serum triglycerides (TG), low-density lipoprotein (LDL), and oxidative stress by modulating mitochondrial lipid metabolism and SIRT1-AMPK pathways . In NAFLD models, TRIC Acid combined with low/mid-dose obeticholic acid (OCA), an FXR agonist, achieves efficacy comparable to high-dose OCA monotherapy while avoiding LDL/HDL dysregulation and lipotoxicity . Mechanistically, this combination downregulates pro-inflammatory (IL-1β) and pro-fibrotic (α-SMA) markers, improving steatohepatitis and fibrosis .
作用機序
Target of Action
The primary target of 10,12-Tricosadiynoic acid is acyl-CoA oxidase-1 (ACOX1) . ACOX1 is a key enzyme involved in the peroxisomal fatty acid beta-oxidation pathway, which is crucial for lipid metabolism .
Mode of Action
This compound acts as a highly specific, selective, and high-affinity inhibitor of ACOX1 . It inhibits ACOX1 activity in a time- and concentration-dependent manner . The inhibition of ACOX1 by this compound-CoA is irreversible .
Biochemical Pathways
The inhibition of ACOX1 by this compound impacts the peroxisomal fatty acid beta-oxidation pathway . This pathway is essential for the breakdown of fatty acids, and its disruption can lead to alterations in lipid metabolism .
Result of Action
The inhibition of ACOX1 by this compound leads to improvements in mitochondrial lipid and reactive oxygen species (ROS) metabolism . This can be beneficial in the treatment of metabolic diseases induced by a high-fat diet or obesity . Inhibition of ACOX1 has been shown to reduce liver lipid and ROS levels, decrease weight gain, and lower serum triglyceride and insulin levels in animal models .
Action Environment
The action of this compound can be influenced by various environmental factors. For instance, diet can play a significant role, as the compound has been shown to be effective in treating metabolic diseases induced by a high-fat diet . .
生物活性
10,12-Tricosadiynoic acid (TCDA), a fatty acid with the chemical formula C23H42O2, is notable for its biological activity as an acyl-CoA oxidase-1 (ACOX1) inhibitor. This compound has gained attention due to its potential therapeutic applications in metabolic diseases, particularly those related to obesity and high-fat diets. The following sections will delve into its biological activity, mechanisms of action, and relevant research findings.
This compound exerts its biological effects primarily through the inhibition of ACOX1, an enzyme crucial for the peroxisomal β-oxidation of very long-chain fatty acids. The inhibition of ACOX1 leads to alterations in lipid metabolism and reactive oxygen species (ROS) generation, which are significant in the context of metabolic disorders.
- Inhibition Characteristics :
Effects on Metabolic Diseases
Research indicates that TCDA can ameliorate conditions induced by high-fat diets by improving mitochondrial lipid metabolism and reducing oxidative stress. In particular:
- Obesity and Metabolic Syndrome : TCDA has shown promise in treating obesity-related metabolic diseases by enhancing mitochondrial function and lipid metabolism .
- Kidney Protection : In studies involving Sirtuin 5-deficient mice, TCDA was used to inhibit ACOX1 activity, leading to improved kidney function and reduced tissue damage in models of acute kidney injury (AKI) induced by ischemia or cisplatin .
Study on Fatty Acid Oxidation
A study published in 2022 demonstrated that TCDA administration significantly suppressed peroxisomal β-oxidation in liver tissues. The results indicated that the compound could modulate cholesterol biosynthesis pathways and influence lipid accumulation in diabetic models .
Parameter | Control Group | TCDA Treatment Group | Effect |
---|---|---|---|
Liver cholesterol content | Increased by 55% | Reduced by TCDA | Inhibition of cholesterol synthesis |
Plasma VLDL-C levels | Increased by 128% | Reduced by TCDA | Lowered lipid levels |
Serum mevalonic acid levels | Increased by 42% | Reduced by TCDA | Indicates decreased cholesterol biosynthesis |
Impact on Adipocyte Function
In adipocyte studies using the 3T3-L1 cell line, TCDA was shown to inhibit peroxisomal fatty acid oxidation effectively. This suggests its role in modulating adipocyte metabolism and potentially influencing fat storage and mobilization .
Summary of Findings
The compound's ability to inhibit ACOX1 presents a significant opportunity for therapeutic interventions in metabolic disorders. Its effects on lipid metabolism are particularly relevant for conditions such as obesity and diabetes, where dysregulated fatty acid oxidation contributes to disease pathology.
科学的研究の応用
Metabolic Disease Treatment
1.1 Mechanism of Action
10,12-Tricosadiynoic acid functions as a highly selective inhibitor of acyl-CoA oxidase-1 (ACOX1), an enzyme involved in fatty acid metabolism. By inhibiting ACOX1, it enhances mitochondrial lipid oxidation and reduces reactive oxygen species (ROS) accumulation, which is particularly beneficial in conditions like obesity and metabolic syndrome .
1.2 Animal Studies
In studies involving male Wistar rats fed a high-fat diet, administration of this compound led to significant improvements in hepatic lipid metabolism. The treatment resulted in:
- Increased mitochondrial fatty acid oxidation.
- Activation of the SIRT1-AMPK signaling pathway.
- Reduction in body weight gain and serum triglyceride levels .
Table 1: Effects of this compound on Metabolic Parameters in Rats
Parameter | Control Group (High-Fat Diet) | Treatment Group (HFD + this compound) |
---|---|---|
Body Weight Gain (g) | 15 | 5 |
Serum Triglycerides (mg/dL) | 250 | 150 |
Hepatic Lipid Content (%) | 30 | 15 |
ROS Levels (µM) | 20 | 10 |
Click Chemistry Applications
This compound contains alkyne functional groups that allow it to participate in copper-catalyzed azide-alkyne cycloaddition reactions. This property makes it a valuable reagent in click chemistry for synthesizing complex molecules and materials .
2.1 Polymer Science
In material science, it has been used to develop acid-responsive polydiacetylene systems. These systems exhibit color changes in response to environmental pH variations, making them suitable for applications in sensors and smart materials .
Case Studies and Research Findings
3.1 Study on Non-Alcoholic Fatty Liver Disease (NAFLD)
A study demonstrated that the combination of this compound with obeticholic acid improved steatohepatitis and liver fibrosis in NAFLD models. The inhibition of ACOX1 was shown to enhance the therapeutic efficacy of obeticholic acid by reducing LDL levels and improving overall liver health .
3.2 Sirtuin Regulation Study
Research indicated that treatment with this compound increased the expression of SIRT1 in liver tissues from high-fat diet-fed rats. This suggests a role for SIRT1 activation in mediating the beneficial effects on lipid metabolism and oxidative stress reduction .
Q & A
Basic Research Questions
Q. What is the molecular mechanism by which 10,12-tricosadiynoic acid inhibits ACOX1 activity?
this compound acts as a prodrug, requiring intracellular conversion to its active CoA form via peroxisomal very long-chain acyl-CoA synthetase (VLACS). The CoA derivative irreversibly inhibits acyl-CoA oxidase-1 (ACOX1), a key enzyme in peroxisomal β-oxidation, by binding with high specificity (KI = 680 nM, kinact = 3.18 min⁻¹). This inhibition disrupts lipid metabolism, reducing ROS generation and improving mitochondrial function in metabolic disease models .
Q. What experimental protocols are recommended for in vitro studies of this compound's effects on lipid metabolism?
For cell-based assays, incubate cells with 500 nM this compound for 24–48 hours. Monitor ACOX1 activity via spectrophotometric assays measuring H₂O₂ production or palmitoyl-CoA oxidation rates. Include controls with VLACS inhibitors (e.g., thioridazine) to confirm the requirement for CoA conjugation. Validate ROS reduction using fluorescent probes (e.g., DCFH-DA) and lipid accumulation via Oil Red O staining .
Q. How should in vivo models be designed to evaluate this compound in obesity-related metabolic disorders?
Use male Wistar rats fed a high-fat diet (HFD) for 8 weeks. Administer 100 µg/kg/day this compound via oral gavage. Key endpoints include:
- Liver metrics : Lipid content (gravimetric analysis), ROS (TBARS assay), and mitochondrial β-oxidation (CPT1 activity).
- Systemic effects : Serum triglycerides, insulin levels, and body weight.
Note: Dose-dependent paradoxes occur in fish models (e.g., Nile tilapia), where lower doses (37.5–150 mg/kg diet) impair β-oxidation and increase hepatic lipid peroxidation .
Advanced Research Questions
Q. How can contradictory data on this compound's dose-response effects between mammalian and fish models be resolved?
Species-specific differences in peroxisomal enzyme regulation may explain disparities. In mammals, SIRT1-AMPK activation dominates, enhancing mitochondrial β-oxidation. In fish, suppressed peroxisomal β-oxidation (via ACOX1 inhibition) may overwhelm compensatory pathways. To reconcile results:
- Conduct cross-species comparative studies with isoform-specific ACOX1 inhibitors.
- Quantify SIRT5 activity, which modulates acetylation-dependent ACOX1 function and is disrupted by this compound in siRNA models .
Q. What strategies optimize the oral bioavailability of this compound for preclinical studies?
Formulate the compound with lipid-based carriers (e.g., micelles or liposomes) to enhance intestinal absorption. Validate bioavailability via LC-MS/MS pharmacokinetic profiling in plasma and liver homogenates. Compare efficacy against intraperitoneal administration to assess first-pass metabolism limitations .
Q. How does this compound interact with SIRT1-AMPK-PPARα signaling in metabolic tissues?
The compound activates SIRT1, deacetylating PPARα and upregulating mitochondrial fatty acid oxidation genes (e.g., CPT1A). Concurrently, AMPK phosphorylation enhances glucose uptake. Use siRNA knockdowns or SIRT1 inhibitors (e.g., EX527) to dissect this pathway. Transcriptomic profiling (RNA-seq) of liver tissue can identify downstream targets .
Q. What analytical methods are critical for characterizing this compound in polymerizable monolayer studies?
For Langmuir-Blodgett film synthesis:
- Use UV-vis spectroscopy to monitor diacetylene polymerization (absorption peak shift from 650 nm to 540 nm upon pH-induced chromatic transition).
- Validate monolayer structure via atomic force microscopy (AFM) and grazing-angle FTIR.
Note: The compound’s amphiphilic properties enable pH-responsive colorimetric sensors, with transitions at pH < 4 or > 10 .
Q. Methodological Considerations
- Data Contradictions : Address interspecies variability by standardizing metabolic endpoints (e.g., β-oxidation rates normalized to tissue weight).
- ROS Quantification : Combine fluorescent probes (e.g., MitoSOX) with enzymatic assays (SOD/catalase activity) to distinguish mitochondrial vs. peroxisomal ROS sources .
- Synthesis & Purity : Source ≥98% pure compound (GC/HPLC-validated) to avoid batch variability. Polymerization studies require monomeric purity >99% to prevent irregular film formation .
類似化合物との比較
FXR Agonists (OCA, GW4064, Cilofexor)
Key Findings :
- TRIC Acid vs. OCA : TRIC Acid combined with low/mid-dose OCA matches high-dose OCA’s efficacy (NASH score reduction: p < 0.001; fibrosis improvement) without elevating LDL .
- TRIC Acid vs.
Mitochondrial β-Oxidation Inhibitors (Etomoxir)
Key Findings :
- TRIC Acid specifically targets peroxisomal β-oxidation, reducing hydrogen peroxide accumulation and oxidative stress without impairing mitochondrial function, unlike etomoxir .
- In vitro, TRIC Acid’s induction of HILPDA (a lipid droplet regulator) is weaker than etomoxir’s, suggesting distinct pathways .
Structural Analogs in Material Science (HDDA, PCDA)
Key Findings :
- TRIC Acid’s diacetylene backbone is structurally analogous to HDDA and PCDA but tailored for therapeutic use via ACOX1 inhibition. Material science analogs prioritize optical properties over metabolic activity .
準備方法
Alkaline Hydrolysis with Copper Catalysis
The most widely documented synthesis of 10,12-tricosadiynoic acid involves alkaline hydrolysis of a propargyl ester precursor under copper(I) catalysis. In a representative procedure, potassium hydroxide (KOH) and methylamine are combined with copper(I) chloride (CuCl) in a tetrahydrofuran (THF)-methanol-water solvent system . The reaction proceeds in two stages:
-
Initial coupling (20°C, 4 hours): The propargyl ester undergoes deprotonation by KOH, forming a copper-acetylide intermediate that facilitates alkyne coupling.
-
Hydrolysis (40°C, 1 hour): The intermediate is hydrolyzed to the carboxylic acid using aqueous methanol.
This method achieves a moderate yield of 48%, with the copper catalyst critical for stabilizing reactive intermediates . Post-reaction purification typically involves extraction with chloroform and filtration to remove polymeric byproducts . Challenges include sensitivity to oxygen, necessitating inert atmospheres, and the need for rigorous temperature control to prevent over-hydrolysis.
Sequential Alkylation of Protected Diynes
A robust alternative, developed to address limitations of direct coupling methods, employs 1,4-bis(trimethylsilyl)-1,3-butadiyne (BTMSBD) as a protected diyne building block . The synthesis involves four stages:
Silyl Protection and Alkylation
BTMSBD is treated with methyl lithium to generate a lithium acetylide, which undergoes alkylation with ω-bromoalkyl halides (e.g., 9-bromo-1-nonanol). This step installs the C10 and C12 alkynes while maintaining regioselectivity .
Deprotection and Acid Formation
The trimethylsilyl (TMS) groups are removed via hydrolysis with potassium fluoride (KF) in dimethylformamide (DMF), yielding a terminal diyne. Subsequent oxidation with Jones reagent (CrO₃/H₂SO₄) converts the alcohol to the carboxylic acid .
Optimization and Yield
Modifications, such as using hexamethylphosphoramide (HMPA) as a co-solvent, improve alkylation efficiency, achieving yields comparable to the copper-catalyzed method (45–48%) . Key advantages include scalability and reduced symmetrical diyne contamination, a common issue in Cadiot-Chodkiewicz approaches.
Cadiot-Chodkiewicz Coupling
While less frequently employed, Cadiot-Chodkiewicz coupling has been explored for synthesizing unsymmetrical diacetylenic acids. This method couples a terminal alkyne with a bromoalkyne in the presence of a copper-amine complex . For this compound, the reaction involves:
-
Step 1: Bromination of 10-undecynoic acid to form 10-bromo-10-undecynoic acid.
-
Step 2: Coupling with 1-dodecyne using CuCl and ethylenediamine.
Despite theoretical appeal, practical limitations include low yields (≤30%) and contamination by symmetrical diynes (e.g., 10,12-docosadiynoic acid) . Consequently, this method is largely superseded by sequential alkylation.
Comparative Analysis of Synthesis Methods
The table below summarizes critical parameters for each method:
Practical Considerations and Industrial Applications
Industrial-scale production favors sequential alkylation due to reproducibility and ease of purification. For laboratory settings, the copper-catalyzed method remains popular for its simplicity. Recent advances focus on solvent optimization; for example, replacing THF with 2-methyltetrahydrofuran (2-MeTHF) improves sustainability without compromising yield .
In pharmaceutical contexts, this compound is utilized in polymerized diacetylene (PDA) vesicles for drug delivery. Its incorporation into vesicles requires dissolution in chloroform followed by filtration (0.45 μm nylon) to eliminate pre-polymerized particles .
特性
IUPAC Name |
tricosa-10,12-diynoic acid | |
---|---|---|
Source | PubChem | |
URL | https://pubchem.ncbi.nlm.nih.gov | |
Description | Data deposited in or computed by PubChem | |
InChI |
InChI=1S/C23H38O2/c1-2-3-4-5-6-7-8-9-10-11-12-13-14-15-16-17-18-19-20-21-22-23(24)25/h2-10,15-22H2,1H3,(H,24,25) | |
Source | PubChem | |
URL | https://pubchem.ncbi.nlm.nih.gov | |
Description | Data deposited in or computed by PubChem | |
InChI Key |
DIEDVCMBPCRJFQ-UHFFFAOYSA-N | |
Source | PubChem | |
URL | https://pubchem.ncbi.nlm.nih.gov | |
Description | Data deposited in or computed by PubChem | |
Canonical SMILES |
CCCCCCCCCCC#CC#CCCCCCCCCC(=O)O | |
Source | PubChem | |
URL | https://pubchem.ncbi.nlm.nih.gov | |
Description | Data deposited in or computed by PubChem | |
Molecular Formula |
C23H38O2 | |
Source | PubChem | |
URL | https://pubchem.ncbi.nlm.nih.gov | |
Description | Data deposited in or computed by PubChem | |
Related CAS |
66990-31-6 | |
Record name | 10,12-Tricosadiynoic acid, homopolymer | |
Source | CAS Common Chemistry | |
URL | https://commonchemistry.cas.org/detail?cas_rn=66990-31-6 | |
Description | CAS Common Chemistry is an open community resource for accessing chemical information. Nearly 500,000 chemical substances from CAS REGISTRY cover areas of community interest, including common and frequently regulated chemicals, and those relevant to high school and undergraduate chemistry classes. This chemical information, curated by our expert scientists, is provided in alignment with our mission as a division of the American Chemical Society. | |
Explanation | The data from CAS Common Chemistry is provided under a CC-BY-NC 4.0 license, unless otherwise stated. | |
DSSTOX Substance ID |
DTXSID40337084 | |
Record name | 10,12-Tricosadiynoic acid | |
Source | EPA DSSTox | |
URL | https://comptox.epa.gov/dashboard/DTXSID40337084 | |
Description | DSSTox provides a high quality public chemistry resource for supporting improved predictive toxicology. | |
Molecular Weight |
346.5 g/mol | |
Source | PubChem | |
URL | https://pubchem.ncbi.nlm.nih.gov | |
Description | Data deposited in or computed by PubChem | |
CAS No. |
66990-30-5 | |
Record name | 10,12-Tricosadiynoic acid | |
Source | EPA DSSTox | |
URL | https://comptox.epa.gov/dashboard/DTXSID40337084 | |
Description | DSSTox provides a high quality public chemistry resource for supporting improved predictive toxicology. | |
Record name | 10,12-Tricosadiynoic Acid | |
Source | European Chemicals Agency (ECHA) | |
URL | https://echa.europa.eu/information-on-chemicals | |
Description | The European Chemicals Agency (ECHA) is an agency of the European Union which is the driving force among regulatory authorities in implementing the EU's groundbreaking chemicals legislation for the benefit of human health and the environment as well as for innovation and competitiveness. | |
Explanation | Use of the information, documents and data from the ECHA website is subject to the terms and conditions of this Legal Notice, and subject to other binding limitations provided for under applicable law, the information, documents and data made available on the ECHA website may be reproduced, distributed and/or used, totally or in part, for non-commercial purposes provided that ECHA is acknowledged as the source: "Source: European Chemicals Agency, http://echa.europa.eu/". Such acknowledgement must be included in each copy of the material. ECHA permits and encourages organisations and individuals to create links to the ECHA website under the following cumulative conditions: Links can only be made to webpages that provide a link to the Legal Notice page. | |
Synthesis routes and methods
Procedure details
Retrosynthesis Analysis
AI-Powered Synthesis Planning: Our tool employs the Template_relevance Pistachio, Template_relevance Bkms_metabolic, Template_relevance Pistachio_ringbreaker, Template_relevance Reaxys, Template_relevance Reaxys_biocatalysis model, leveraging a vast database of chemical reactions to predict feasible synthetic routes.
One-Step Synthesis Focus: Specifically designed for one-step synthesis, it provides concise and direct routes for your target compounds, streamlining the synthesis process.
Accurate Predictions: Utilizing the extensive PISTACHIO, BKMS_METABOLIC, PISTACHIO_RINGBREAKER, REAXYS, REAXYS_BIOCATALYSIS database, our tool offers high-accuracy predictions, reflecting the latest in chemical research and data.
Strategy Settings
Precursor scoring | Relevance Heuristic |
---|---|
Min. plausibility | 0.01 |
Model | Template_relevance |
Template Set | Pistachio/Bkms_metabolic/Pistachio_ringbreaker/Reaxys/Reaxys_biocatalysis |
Top-N result to add to graph | 6 |
Feasible Synthetic Routes
試験管内研究製品の免責事項と情報
BenchChemで提示されるすべての記事および製品情報は、情報提供を目的としています。BenchChemで購入可能な製品は、生体外研究のために特別に設計されています。生体外研究は、ラテン語の "in glass" に由来し、生物体の外で行われる実験を指します。これらの製品は医薬品または薬として分類されておらず、FDAから任何の医療状態、病気、または疾患の予防、治療、または治癒のために承認されていません。これらの製品を人間または動物に体内に導入する形態は、法律により厳格に禁止されています。これらのガイドラインに従うことは、研究と実験において法的および倫理的な基準の遵守を確実にするために重要です。