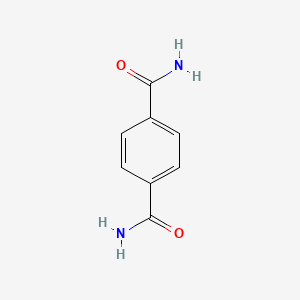
テレフタルアミド
概要
説明
Terephthalamide is an organic compound derived from terephthalic acid. It is a type of aromatic amide, characterized by the presence of an amide group attached to a benzene ring. Terephthalamide is known for its high thermal stability and mechanical strength, making it a valuable material in various industrial applications.
科学的研究の応用
Advanced Materials and Fibers
Poly(p-phenylene terephthalamide) (PPTA) , commonly known as Kevlar, is a notable derivative of terephthalamide. It is renowned for its exceptional mechanical properties and thermal stability, making it suitable for high-performance applications:
- Ballistic Protection : Kevlar fibers are extensively used in body armor and protective gear. Their lightweight yet strong characteristics provide crucial protection against ballistic threats, significantly enhancing safety for military and law enforcement personnel .
- Industrial Applications : Beyond personal protection, PPTA is utilized in manufacturing ropes, cables, and protective clothing. Its resistance to heat and chemicals allows it to function effectively in harsh environments .
- Sporting Goods : The material's strength-to-weight ratio has led to its incorporation in sports equipment such as tennis racquets and bicycles, where performance and durability are paramount .
Biodegradation Studies
Recent research has explored the biodegradability of terephthalamide, revealing insights into its environmental impact:
- Microbial Degradation : A study identified microbial communities capable of degrading terephthalamide in natural environments. This discovery is significant as it suggests potential bioremediation strategies for terephthalamide contamination . The findings indicate that while terephthalamide was previously considered non-biodegradable, specific microbial consortia can effectively break it down, highlighting the importance of ecological approaches to managing synthetic pollutants.
- Environmental Implications : Understanding the biodegradation pathways for terephthalamide can inform waste management practices and contribute to sustainable development goals by mitigating the environmental footprint of synthetic polymers .
Biomedical Applications
Terephthalamide derivatives have been investigated for their potential in biomedical fields:
- Drug Delivery Systems : Research has shown that terephthalamide-based polymers can be utilized as scaffolds for drug delivery and tissue engineering. Their biocompatibility and ability to form hydrogels make them suitable for controlled release applications .
- Synthetic Biomaterials : Terephthalamide's properties have led to its use in developing synthetic biodegradable polymers. These materials are increasingly employed in clinical settings for drug delivery systems targeting cancer therapies, demonstrating their relevance in modern medicine .
Case Study 1: Kevlar in Military Applications
A comprehensive analysis of Kevlar's use in military body armor illustrates its life-saving capabilities. The material's design allows it to absorb and disperse impact forces effectively, reducing injury rates among personnel in combat situations.
Case Study 2: Biodegradation Research
The study on microbial degradation of terephthalamide involved sampling from diverse environments to identify effective degrading microorganisms. The results indicated significant variations in microbial communities' abilities to degrade terephthalamide based on environmental conditions, showcasing the complexity of biodegradation processes.
作用機序
Target of Action
Terephthalamide is primarily involved in the recycling of polyethylene terephthalate (PET), a common plastic material . Its primary targets are the PET molecules, which it helps to break down into simpler components .
Mode of Action
Terephthalamide interacts with PET molecules through a process known as aminolysis . This process involves the breaking of PET into its monomers, ethylene glycol (EG) and terephthalic acid (TPA), with the help of catalysts . The resulting monomers can then be transformed into new materials .
Biochemical Pathways
The biochemical pathways involved in the action of Terephthalamide primarily revolve around the degradation and recycling of PET . Microbial cell factories provide a promising approach to degrade PET and convert its monomers, EG and TPA, into value-added compounds . The degradation of PET monomers is facilitated by microbes and metabolic pathways .
Pharmacokinetics
The preparation of poly(p-phenylene terephthalamide) (ppta) involves a two-step method, which includes fast conversion of reactive groups and further chain growth .
Result of Action
The result of Terephthalamide’s action is the transformation of PET into its monomers, EG and TPA . These monomers can then be used to create new materials, effectively recycling the original PET . This process helps to minimize overall crude oil-based materials consumption and decrease greenhouse gas emissions .
Action Environment
The action of Terephthalamide is influenced by various environmental factors. For instance, the efficiency of PET depolymerization can be affected by the presence of certain catalysts . Furthermore, the process of PET recycling is of great significance for solving environmental problems and reducing the plastic industry’s dependence on petrochemical resources .
生化学分析
Biochemical Properties
Terephthalamide plays a significant role in biochemical reactions, particularly in the degradation and recycling of PET. It interacts with various enzymes, such as PET hydrolases, which catalyze the breakdown of PET into its monomers, including terephthalamide. These interactions are crucial for the efficient recycling of PET and the reduction of plastic waste .
Cellular Effects
Terephthalamide influences various cellular processes, including cell signaling pathways, gene expression, and cellular metabolism. It has been observed to affect the expression of genes involved in stress responses and metabolic pathways. Additionally, terephthalamide can modulate cell signaling pathways, leading to changes in cellular functions and metabolic activities .
Molecular Mechanism
At the molecular level, terephthalamide exerts its effects through binding interactions with specific biomolecules. It can inhibit or activate enzymes involved in PET degradation, thereby influencing the overall efficiency of the recycling process. Terephthalamide also affects gene expression by interacting with transcription factors and other regulatory proteins .
Temporal Effects in Laboratory Settings
In laboratory settings, the effects of terephthalamide change over time. The compound’s stability and degradation are critical factors that influence its long-term effects on cellular function. Studies have shown that terephthalamide remains stable under specific conditions, but its degradation products can have varying impacts on cells and tissues .
Dosage Effects in Animal Models
The effects of terephthalamide vary with different dosages in animal models. At low doses, it may have minimal impact on cellular functions, while higher doses can lead to toxic or adverse effects. Threshold effects have been observed, indicating that there is a specific dosage range within which terephthalamide exerts its optimal effects without causing harm .
Metabolic Pathways
Terephthalamide is involved in several metabolic pathways, including those related to PET degradation. It interacts with enzymes such as PET hydrolases and other cofactors that facilitate the breakdown of PET into its monomers. These interactions can affect metabolic flux and metabolite levels, influencing the overall efficiency of PET recycling .
Transport and Distribution
Within cells and tissues, terephthalamide is transported and distributed through specific transporters and binding proteins. These interactions determine its localization and accumulation in different cellular compartments. The transport and distribution of terephthalamide are crucial for its effective functioning in biochemical reactions .
Subcellular Localization
Terephthalamide’s subcellular localization is influenced by targeting signals and post-translational modifications. These factors direct the compound to specific compartments or organelles, where it can exert its effects on cellular functions. Understanding the subcellular localization of terephthalamide is essential for elucidating its role in biochemical processes .
準備方法
Synthetic Routes and Reaction Conditions: Terephthalamide can be synthesized through the polycondensation reaction of terephthaloyl chloride with p-phenylenediamine. This reaction typically occurs in a solvent such as N-methyl-2-pyrrolidone (NMP) under an inert atmosphere to prevent oxidation. The reaction is exothermic and requires careful control of temperature and reaction time to achieve high molecular weight polymers .
Industrial Production Methods: In industrial settings, terephthalamide is produced using a continuous polymerization process. The reactants are fed into a reactor where they undergo polycondensation at elevated temperatures. The resulting polymer is then extruded, cooled, and cut into pellets for further processing. This method ensures consistent quality and high production efficiency .
化学反応の分析
Types of Reactions: Terephthalamide undergoes various chemical reactions, including:
Oxidation: Terephthalamide can be oxidized to form terephthalic acid.
Reduction: Reduction of terephthalamide can yield p-phenylenediamine.
Substitution: The amide group in terephthalamide can be substituted with other functional groups under appropriate conditions.
Common Reagents and Conditions:
Oxidation: Common oxidizing agents include potassium permanganate and nitric acid.
Reduction: Reducing agents such as lithium aluminum hydride are used.
Substitution: Substitution reactions often require catalysts and specific solvents to facilitate the reaction.
Major Products Formed:
Oxidation: Terephthalic acid.
Reduction: p-Phenylenediamine.
Substitution: Various substituted aromatic amides depending on the substituent introduced.
類似化合物との比較
Poly(p-phenylene terephthalamide) (Kevlar): Known for its exceptional strength and thermal stability, Kevlar is widely used in bulletproof vests and other protective gear.
Poly(m-phenylene isophthalamide): Another aromatic amide with high thermal stability, used in heat-resistant fabrics and insulation materials.
Uniqueness of Terephthalamide: Terephthalamide stands out due to its balanced properties of mechanical strength, thermal stability, and chemical resistance. Its ability to form high-performance polymers makes it a versatile material for various advanced applications .
生物活性
Terephthalamide, a compound derived from terephthalic acid, has garnered attention in various fields of research due to its diverse biological activities. This article provides a comprehensive overview of the biological activity of terephthalamide, including its antibacterial, antifungal, and potential anticancer properties. The synthesis methods and structure-activity relationships (SAR) will also be discussed, supported by relevant case studies and data tables.
Synthesis and Structural Characteristics
Terephthalamide can be synthesized through various methods, often involving the reaction of terephthalic acid derivatives with amines. The structural characteristics of terephthalamide derivatives significantly influence their biological activities. For instance, modifications such as alkyl chain length in thiourea derivatives have been shown to enhance antibacterial properties by increasing lipophilicity, which aids in disrupting microbial cell walls .
Antibacterial Activity
Recent studies have highlighted the antibacterial efficacy of terephthalamide derivatives against Gram-negative bacteria. A notable example is the synthesis of bis(thiourea) derivatives of terephthalamide, which demonstrated significant bacteriostatic activity against Escherichia coli. The minimum inhibitory concentration (MIC) values for these compounds were reported as follows:
Compound | MIC (µg/mL) |
---|---|
1,4-bis(decoxyphenyl)carbamothioyl-terephthalamide (n=10) | 135 |
1,4-bis(decoxyphenyl)carbamothioyl-terephthalamide (n=12) | 145 |
The presence of thiourea moieties was crucial for this activity as they can interact with bacterial surface groups, enhancing their antimicrobial effects .
Antifungal Activity
Terephthalamide derivatives have also shown promising antifungal properties. A study evaluated the antifungal activity of a novel class of cationic assemblies based on terephthalamide against Candida albicans and Cryptococcus neoformans. The results indicated that these assemblies caused significant damage to fungal cell membranes, leading to cell lysis and nucleic acid release in a dose-dependent manner. The following table summarizes the antifungal activity:
Compound | Target Organism | Observed Effect |
---|---|---|
Terephthalamide-based assembly | C. albicans | Membrane damage and cell lysis |
Terephthalamide-based assembly | C. neoformans | Release of cytoplast and nucleic acids |
These findings suggest that terephthalamide derivatives can effectively disrupt fungal cell integrity, making them potential candidates for antifungal drug development .
Anticancer Potential
The anticancer properties of terephthalamide have been explored through its ability to disrupt protein interactions critical for cancer cell survival. Specifically, certain terephthalamide derivatives have been identified as mimetics of helical peptides that can inhibit the interaction between Bcl-x(L) and Bak proteins, which are involved in apoptosis regulation. This mechanism positions terephthalamide as a potential therapeutic agent in cancer treatment .
Case Studies
- Antibacterial Efficacy : A study focused on synthesizing novel 1,4-bis(decoxyphenyl)carbamothioyl-terephthalamide derivatives demonstrated their effectiveness against E. coli, highlighting the role of structural modifications in enhancing antibacterial properties .
- Antifungal Mechanism : Research into cationic assemblies based on terephthalamide revealed their capacity to induce significant morphological changes in fungal cells, indicating their potential as antifungal agents .
- Cancer Therapeutics : Investigations into the interaction between terephthalamide derivatives and apoptotic proteins provided insights into their possible applications in cancer therapy, showcasing their dual role as both structural mimetics and functional inhibitors .
特性
IUPAC Name |
benzene-1,4-dicarboxamide | |
---|---|---|
Source | PubChem | |
URL | https://pubchem.ncbi.nlm.nih.gov | |
Description | Data deposited in or computed by PubChem | |
InChI |
InChI=1S/C8H8N2O2/c9-7(11)5-1-2-6(4-3-5)8(10)12/h1-4H,(H2,9,11)(H2,10,12) | |
Source | PubChem | |
URL | https://pubchem.ncbi.nlm.nih.gov | |
Description | Data deposited in or computed by PubChem | |
InChI Key |
MHSKRLJMQQNJNC-UHFFFAOYSA-N | |
Source | PubChem | |
URL | https://pubchem.ncbi.nlm.nih.gov | |
Description | Data deposited in or computed by PubChem | |
Canonical SMILES |
C1=CC(=CC=C1C(=O)N)C(=O)N | |
Source | PubChem | |
URL | https://pubchem.ncbi.nlm.nih.gov | |
Description | Data deposited in or computed by PubChem | |
Molecular Formula |
C8H8N2O2 | |
Source | PubChem | |
URL | https://pubchem.ncbi.nlm.nih.gov | |
Description | Data deposited in or computed by PubChem | |
Related CAS |
120253-63-6 | |
Record name | Polyterephthalamide | |
Source | CAS Common Chemistry | |
URL | https://commonchemistry.cas.org/detail?cas_rn=120253-63-6 | |
Description | CAS Common Chemistry is an open community resource for accessing chemical information. Nearly 500,000 chemical substances from CAS REGISTRY cover areas of community interest, including common and frequently regulated chemicals, and those relevant to high school and undergraduate chemistry classes. This chemical information, curated by our expert scientists, is provided in alignment with our mission as a division of the American Chemical Society. | |
Explanation | The data from CAS Common Chemistry is provided under a CC-BY-NC 4.0 license, unless otherwise stated. | |
DSSTOX Substance ID |
DTXSID2051976 | |
Record name | 1,4-Benzenedicarboxamide | |
Source | EPA DSSTox | |
URL | https://comptox.epa.gov/dashboard/DTXSID2051976 | |
Description | DSSTox provides a high quality public chemistry resource for supporting improved predictive toxicology. | |
Molecular Weight |
164.16 g/mol | |
Source | PubChem | |
URL | https://pubchem.ncbi.nlm.nih.gov | |
Description | Data deposited in or computed by PubChem | |
CAS No. |
3010-82-0 | |
Record name | Terephthalamide | |
Source | CAS Common Chemistry | |
URL | https://commonchemistry.cas.org/detail?cas_rn=3010-82-0 | |
Description | CAS Common Chemistry is an open community resource for accessing chemical information. Nearly 500,000 chemical substances from CAS REGISTRY cover areas of community interest, including common and frequently regulated chemicals, and those relevant to high school and undergraduate chemistry classes. This chemical information, curated by our expert scientists, is provided in alignment with our mission as a division of the American Chemical Society. | |
Explanation | The data from CAS Common Chemistry is provided under a CC-BY-NC 4.0 license, unless otherwise stated. | |
Record name | Terephthaldiamide | |
Source | ChemIDplus | |
URL | https://pubchem.ncbi.nlm.nih.gov/substance/?source=chemidplus&sourceid=0003010820 | |
Description | ChemIDplus is a free, web search system that provides access to the structure and nomenclature authority files used for the identification of chemical substances cited in National Library of Medicine (NLM) databases, including the TOXNET system. | |
Record name | Terephthalamide | |
Source | DTP/NCI | |
URL | https://dtp.cancer.gov/dtpstandard/servlet/dwindex?searchtype=NSC&outputformat=html&searchlist=56420 | |
Description | The NCI Development Therapeutics Program (DTP) provides services and resources to the academic and private-sector research communities worldwide to facilitate the discovery and development of new cancer therapeutic agents. | |
Explanation | Unless otherwise indicated, all text within NCI products is free of copyright and may be reused without our permission. Credit the National Cancer Institute as the source. | |
Record name | 1,4-Benzenedicarboxamide | |
Source | EPA Chemicals under the TSCA | |
URL | https://www.epa.gov/chemicals-under-tsca | |
Description | EPA Chemicals under the Toxic Substances Control Act (TSCA) collection contains information on chemicals and their regulations under TSCA, including non-confidential content from the TSCA Chemical Substance Inventory and Chemical Data Reporting. | |
Record name | 1,4-Benzenedicarboxamide | |
Source | EPA DSSTox | |
URL | https://comptox.epa.gov/dashboard/DTXSID2051976 | |
Description | DSSTox provides a high quality public chemistry resource for supporting improved predictive toxicology. | |
Record name | TEREPHTHALDIAMIDE | |
Source | FDA Global Substance Registration System (GSRS) | |
URL | https://gsrs.ncats.nih.gov/ginas/app/beta/substances/YAY9TC6F38 | |
Description | The FDA Global Substance Registration System (GSRS) enables the efficient and accurate exchange of information on what substances are in regulated products. Instead of relying on names, which vary across regulatory domains, countries, and regions, the GSRS knowledge base makes it possible for substances to be defined by standardized, scientific descriptions. | |
Explanation | Unless otherwise noted, the contents of the FDA website (www.fda.gov), both text and graphics, are not copyrighted. They are in the public domain and may be republished, reprinted and otherwise used freely by anyone without the need to obtain permission from FDA. Credit to the U.S. Food and Drug Administration as the source is appreciated but not required. | |
Synthesis routes and methods I
Procedure details
Synthesis routes and methods II
Procedure details
Retrosynthesis Analysis
AI-Powered Synthesis Planning: Our tool employs the Template_relevance Pistachio, Template_relevance Bkms_metabolic, Template_relevance Pistachio_ringbreaker, Template_relevance Reaxys, Template_relevance Reaxys_biocatalysis model, leveraging a vast database of chemical reactions to predict feasible synthetic routes.
One-Step Synthesis Focus: Specifically designed for one-step synthesis, it provides concise and direct routes for your target compounds, streamlining the synthesis process.
Accurate Predictions: Utilizing the extensive PISTACHIO, BKMS_METABOLIC, PISTACHIO_RINGBREAKER, REAXYS, REAXYS_BIOCATALYSIS database, our tool offers high-accuracy predictions, reflecting the latest in chemical research and data.
Strategy Settings
Precursor scoring | Relevance Heuristic |
---|---|
Min. plausibility | 0.01 |
Model | Template_relevance |
Template Set | Pistachio/Bkms_metabolic/Pistachio_ringbreaker/Reaxys/Reaxys_biocatalysis |
Top-N result to add to graph | 6 |
Feasible Synthetic Routes
試験管内研究製品の免責事項と情報
BenchChemで提示されるすべての記事および製品情報は、情報提供を目的としています。BenchChemで購入可能な製品は、生体外研究のために特別に設計されています。生体外研究は、ラテン語の "in glass" に由来し、生物体の外で行われる実験を指します。これらの製品は医薬品または薬として分類されておらず、FDAから任何の医療状態、病気、または疾患の予防、治療、または治癒のために承認されていません。これらの製品を人間または動物に体内に導入する形態は、法律により厳格に禁止されています。これらのガイドラインに従うことは、研究と実験において法的および倫理的な基準の遵守を確実にするために重要です。