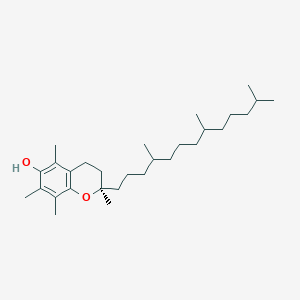
(2R)-2,5,7,8-テトラメチル-2-(4,8,12-トリメチルトリデシル)-3,4-ジヒドロクロメン-6-オール
説明
(2R)-2,5,7,8-Tetramethyl-2-(4,8,12-trimethyltridecyl)-3,4-dihydrochromen-6-ol is a natural product found in Silybum marianum, Jacobaea alpina, and Chlorella vulgaris with data available.
科学的研究の応用
Nutritional Supplementation
Antioxidant Properties
The compound is recognized for its antioxidant capabilities , which help neutralize free radicals in the body. This property is crucial for preventing oxidative stress-related diseases such as cancer and cardiovascular diseases. Research indicates that tocopherols can enhance immune function and may reduce the risk of chronic diseases .
Dietary Sources
Tocopherols are naturally found in various foods including nuts, seeds, and green leafy vegetables. Supplementation with (2R)-2,5,7,8-tetramethyl-2-(4,8,12-trimethyltridecyl)-3,4-dihydrochromen-6-ol can thus be beneficial for individuals lacking sufficient dietary intake .
Cosmetic and Personal Care Products
Skin Protection and Anti-Aging
In cosmetics, this compound is frequently included due to its ability to protect skin cells from UV radiation and environmental pollutants. It helps to prevent premature aging by reducing lipid peroxidation and maintaining skin elasticity .
Moisturizing Agent
The compound acts as an effective moisturizer that enhances the skin's smoothness and elasticity. It is commonly used in formulations for lotions, creams, and sunscreens to provide both hydration and protective benefits .
Pharmaceutical Applications
Therapeutic Uses
Research has shown that tocopherols can play a role in the treatment of specific conditions such as Alzheimer's disease and other neurodegenerative disorders due to their neuroprotective effects . Studies indicate that supplementation may improve cognitive function and slow disease progression.
Drug Formulation
The compound is also utilized in drug formulations as a stabilizer for other active ingredients due to its antioxidant properties. This helps enhance the shelf life and effectiveness of pharmaceutical products .
Food Industry
Preservative Agent
In food products, (2R)-2,5,7,8-Tetramethyl-2-(4,8,12-trimethyltridecyl)-3,4-dihydrochromen-6-ol serves as a natural preservative. Its antioxidant properties help extend the shelf life of oils and fats by preventing rancidity .
Case Studies
作用機序
Target of Action
The primary targets of (+)-alpha-Tocopherol are reactive oxygen species (ROS) . ROS are chemically reactive molecules containing oxygen, which are produced as a natural byproduct of the normal metabolism of oxygen. They play important roles in cell signaling and homeostasis, but during times of environmental stress, ROS levels can increase dramatically, leading to significant damage to cell structures .
Mode of Action
(+)-alpha-Tocopherol interacts with its targets by acting as an antioxidant . It neutralizes ROS by donating a hydrogen atom from the hydroxyl group on the chromanol ring to the free radical . This results in the formation of a relatively stable, unreactive tocopheryl radical, which can be reduced back to tocopherol by ascorbate or other reducing agents .
Biochemical Pathways
The compound affects the antioxidant defense system . By neutralizing ROS, it prevents oxidative stress, a state characterized by an imbalance between the production of ROS and the ability of the body to counteract or detoxify their harmful effects through neutralization by antioxidants . This helps maintain the integrity of cells and enhances the body’s ability to fight off infections and diseases .
Pharmacokinetics
The pharmacokinetics of (+)-alpha-Tocopherol involve its absorption, distribution, metabolism, and excretion (ADME). After oral administration, it is absorbed in the small intestine and incorporated into chylomicrons, which are lipoprotein particles that transport dietary lipids from the intestines to other locations in the body . The compound is then distributed throughout the body, with the liver being the main organ for its storage . It is metabolized primarily in the liver and excreted in the bile .
Result of Action
The molecular and cellular effects of (+)-alpha-Tocopherol’s action include the protection of cell membranes from oxidative damage . By neutralizing ROS, it prevents lipid peroxidation, a process in which free radicals “steal” electrons from the lipids in cell membranes, resulting in cell damage . This helps maintain the integrity of cells and enhances the body’s ability to fight off infections and diseases .
Action Environment
Environmental factors can influence the action, efficacy, and stability of (+)-alpha-Tocopherol. For instance, exposure to ultraviolet (UV) radiation can increase the production of ROS, thereby increasing the demand for antioxidants like (+)-alpha-Tocopherol . Additionally, factors such as diet and lifestyle can affect the body’s antioxidant status and the bioavailability of (+)-alpha-Tocopherol .
生化学分析
Biochemical Properties
(+)-alpha-Tocopherol plays a crucial role in various biochemical reactions. It acts as an antioxidant, reducing oxidative stress by scavenging peroxy radicals of polyunsaturated fatty acids or by reacting with singlet oxygen and other reactive oxygen species (ROS) . The antioxidant activity of (+)-alpha-Tocopherol is higher than other tocopherols, meaning less (+)-alpha-Tocopherol is needed for maximum antioxidant protection .
Cellular Effects
The cellular effects of (+)-alpha-Tocopherol are profound. It has been shown to improve cytotoxicity and increase the intracellular accumulation of certain drugs in cancer cells . It also influences cell function by regulating cellular signaling, cell proliferation, and gene expression .
Molecular Mechanism
At the molecular level, (+)-alpha-Tocopherol exerts its effects through various mechanisms. It donates a hydrogen atom from the hydroxyl group on the chromanol ring, resulting in a “tocopherol radical” formation. These radicals are resonance stabilized within the chromanol ring and do not propagate the chain reactions . It also interacts with biomolecules, inhibiting or activating enzymes, and causing changes in gene expression .
Temporal Effects in Laboratory Settings
In laboratory settings, the effects of (+)-alpha-Tocopherol can change over time. It has been observed that the compound exhibits a concentration- and time-dependent cytotoxicity
Dosage Effects in Animal Models
In animal models, the effects of (+)-alpha-Tocopherol can vary with different dosages. For instance, in a study involving mice, it was found that the dosage of (+)-alpha-Tocopherol could be higher than 30 mg/kg with an inhibition rate of 71.2%, which is 1.57-fold higher than that treated with a control .
Metabolic Pathways
(+)-alpha-Tocopherol is involved in various metabolic pathways. It is a crucial part of the vitamin E metabolic pathway, acting as an essential cofactor for certain enzymes
Transport and Distribution
(+)-alpha-Tocopherol is transported and distributed within cells and tissues through specific transporters and binding proteins . It is lipid-soluble, allowing it to be incorporated into cell membranes and transported to various parts of the cell.
Subcellular Localization
The subcellular localization of (+)-alpha-Tocopherol is primarily in the cell membrane due to its lipid-soluble nature . It can also be found in other organelles like mitochondria and endoplasmic reticulum where it performs its antioxidant functions .
生物活性
(2R)-2,5,7,8-Tetramethyl-2-(4,8,12-trimethyltridecyl)-3,4-dihydrochromen-6-ol, commonly known as D-alpha-tocotrienol , is a member of the vitamin E family and is recognized for its significant biological activities. This compound is primarily found in various plant oils and is noted for its antioxidant properties. The molecular formula for this compound is with a molecular weight of approximately 430.71 g/mol.
Property | Value |
---|---|
Molecular Formula | C29H50O2 |
Molecular Weight | 430.71 g/mol |
Boiling Point | 541.7 °C (predicted) |
Density | 0.960 g/cm³ (predicted) |
pKa | 11.40 (predicted) |
Storage Temperature | -20 °C |
Antioxidant Properties
D-alpha-tocotrienol exhibits potent antioxidant activity, which helps in neutralizing free radicals and reducing oxidative stress in cells. This property is crucial in preventing cellular damage and has implications in aging and chronic diseases such as cancer and cardiovascular diseases.
Anti-Cancer Effects
Research has indicated that tocotrienols can inhibit cancer cell proliferation and induce apoptosis in various cancer types, including breast, prostate, and colon cancers. A study demonstrated that tocotrienols can downregulate the expression of certain oncogenes while upregulating tumor suppressor genes.
Cardiovascular Health
Tocotrienols have been shown to improve cardiovascular health by reducing cholesterol levels and preventing the oxidation of low-density lipoprotein (LDL). This action helps in mitigating the risk of atherosclerosis and other heart-related conditions.
Neuroprotective Effects
Studies have highlighted the neuroprotective effects of tocotrienols against neurodegenerative diseases such as Alzheimer's disease. They may help in preserving cognitive function by reducing oxidative stress and inflammation in neuronal tissues.
Case Studies
- Breast Cancer Study : A clinical trial involving breast cancer patients showed that supplementation with tocotrienols resulted in a significant reduction in tumor size compared to control groups. The study attributed this effect to the induction of apoptosis and inhibition of angiogenesis.
- Cardiovascular Health : In a cohort study examining the effects of tocotrienol-rich palm oil on hypercholesterolemic patients, results indicated a marked decrease in total cholesterol levels and improved lipid profiles after 12 weeks of supplementation.
- Neurodegeneration : A randomized controlled trial focusing on elderly participants found that those receiving tocotrienol supplements exhibited better cognitive performance on memory tests compared to those receiving placebo.
The biological activities of D-alpha-tocotrienol can be attributed to several mechanisms:
- Antioxidant Activity : Tocotrienols scavenge free radicals due to their unique chemical structure that allows them to donate electrons without becoming reactive themselves.
- Gene Expression Modulation : Tocotrienols can influence gene expression related to cell survival and apoptosis pathways.
- Inflammation Reduction : They inhibit pro-inflammatory cytokines and enzymes such as COX-2, contributing to their anti-inflammatory effects.
特性
IUPAC Name |
(2R)-2,5,7,8-tetramethyl-2-(4,8,12-trimethyltridecyl)-3,4-dihydrochromen-6-ol | |
---|---|---|
Source | PubChem | |
URL | https://pubchem.ncbi.nlm.nih.gov | |
Description | Data deposited in or computed by PubChem | |
InChI |
InChI=1S/C29H50O2/c1-20(2)12-9-13-21(3)14-10-15-22(4)16-11-18-29(8)19-17-26-25(7)27(30)23(5)24(6)28(26)31-29/h20-22,30H,9-19H2,1-8H3/t21?,22?,29-/m1/s1 | |
Source | PubChem | |
URL | https://pubchem.ncbi.nlm.nih.gov | |
Description | Data deposited in or computed by PubChem | |
InChI Key |
GVJHHUAWPYXKBD-UQIPPQJZSA-N | |
Source | PubChem | |
URL | https://pubchem.ncbi.nlm.nih.gov | |
Description | Data deposited in or computed by PubChem | |
Canonical SMILES |
CC1=C(C2=C(CCC(O2)(C)CCCC(C)CCCC(C)CCCC(C)C)C(=C1O)C)C | |
Source | PubChem | |
URL | https://pubchem.ncbi.nlm.nih.gov | |
Description | Data deposited in or computed by PubChem | |
Isomeric SMILES |
CC1=C(C2=C(CC[C@@](O2)(C)CCCC(C)CCCC(C)CCCC(C)C)C(=C1O)C)C | |
Source | PubChem | |
URL | https://pubchem.ncbi.nlm.nih.gov | |
Description | Data deposited in or computed by PubChem | |
Molecular Formula |
C29H50O2 | |
Source | PubChem | |
URL | https://pubchem.ncbi.nlm.nih.gov | |
Description | Data deposited in or computed by PubChem | |
Molecular Weight |
430.7 g/mol | |
Source | PubChem | |
URL | https://pubchem.ncbi.nlm.nih.gov | |
Description | Data deposited in or computed by PubChem | |
Retrosynthesis Analysis
AI-Powered Synthesis Planning: Our tool employs the Template_relevance Pistachio, Template_relevance Bkms_metabolic, Template_relevance Pistachio_ringbreaker, Template_relevance Reaxys, Template_relevance Reaxys_biocatalysis model, leveraging a vast database of chemical reactions to predict feasible synthetic routes.
One-Step Synthesis Focus: Specifically designed for one-step synthesis, it provides concise and direct routes for your target compounds, streamlining the synthesis process.
Accurate Predictions: Utilizing the extensive PISTACHIO, BKMS_METABOLIC, PISTACHIO_RINGBREAKER, REAXYS, REAXYS_BIOCATALYSIS database, our tool offers high-accuracy predictions, reflecting the latest in chemical research and data.
Strategy Settings
Precursor scoring | Relevance Heuristic |
---|---|
Min. plausibility | 0.01 |
Model | Template_relevance |
Template Set | Pistachio/Bkms_metabolic/Pistachio_ringbreaker/Reaxys/Reaxys_biocatalysis |
Top-N result to add to graph | 6 |
Feasible Synthetic Routes
Q1: How does the structure of RRR-α-tocopherol influence its bioavailability compared to other forms of vitamin E?
A1: [] A study investigated the bioavailability of RRR-α-tocopherol from different formulations, including soft elastic gelatin capsules (SEG) and tablets. The research showed that RRR-α-tocopherol absorption was significantly enhanced when delivered in an Aquabiosorb SEG capsule compared to a modified standard-SEG capsule or a commercial tablet. This suggests that the formulation, particularly the use of a surfactant vehicle in the SEG capsule, positively impacts the wetting properties and consequently, the bioavailability of RRR-α-tocopherol. [Dosage form and formulation effects on the bioavailability of vitamin E, riboflavin, and vitamin B-6 from multivitamin preparations.]
Q2: Are there differences in how the body utilizes natural versus synthetic forms of vitamin E?
A2: [] Although chemically similar, natural food sources provide vitamin E in a complex matrix alongside other nutrients. These additional factors can influence absorption and utilization in ways not fully replicated by isolated synthetic forms. [The Truth About Vitamins in Supplements.] Additionally, [] research comparing D-alpha-tocopherol to its synthetic racemic counterpart (DL-alpha-tocopherol) found that the natural form led to faster and higher plasma concentrations, suggesting superior bioavailability and potentially different metabolic handling within the body. [Blood plasma and tissue concentrations of vitamin E in beef cattle as influenced by supplementation of various tocopherol compounds.]
Q3: Beyond its antioxidant properties, does RRR-α-tocopherol exhibit any other biological activities?
A3: [] Research has shown that RRR-α-tocopheryl succinate (VES), a derivative of RRR-α-tocopherol, can inhibit the proliferation of chicken T cells when stimulated with mitogens like concanavalin A and phytohemagglutinin. Interestingly, this effect appears distinct from VES's antioxidant properties, as other forms of vitamin E and even known antioxidants did not demonstrate this inhibitory activity. This suggests a unique mechanism of action for VES in modulating immune cell responses. [RRR-alpha-tocopheryl succinate inhibition of lectin-induced T cell proliferation.]
Q4: How do researchers differentiate between various forms of vitamin E in biological samples?
A4: [] High-performance liquid chromatography (HPLC) is a commonly used technique to separate and identify different forms of vitamin E, including alpha-tocopherol, based on their unique chemical properties and interactions with the chromatographic column. Confirmation of the specific form can be achieved by comparing the retention times of the separated compounds with known standards. Furthermore, mass spectrometry is employed to determine the mass-to-charge ratio of the analyzed molecules, offering definitive identification of the specific vitamin E form present in the sample. [Blood plasma and tissue concentrations of vitamin E in beef cattle as influenced by supplementation of various tocopherol compounds.]
試験管内研究製品の免責事項と情報
BenchChemで提示されるすべての記事および製品情報は、情報提供を目的としています。BenchChemで購入可能な製品は、生体外研究のために特別に設計されています。生体外研究は、ラテン語の "in glass" に由来し、生物体の外で行われる実験を指します。これらの製品は医薬品または薬として分類されておらず、FDAから任何の医療状態、病気、または疾患の予防、治療、または治癒のために承認されていません。これらの製品を人間または動物に体内に導入する形態は、法律により厳格に禁止されています。これらのガイドラインに従うことは、研究と実験において法的および倫理的な基準の遵守を確実にするために重要です。