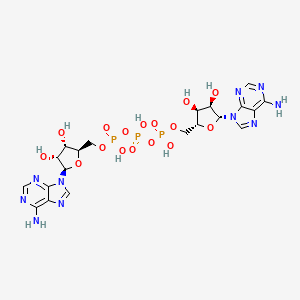
Diadenosine triphosphate
説明
P(1),P(3)-bis(5'-adenosyl) triphosphate is a diadenosyl triphosphate having the two 5'-adenosyl residues attached at the P(1)- and P(3)-positions. It has a role as a mouse metabolite. It is a conjugate acid of a P(1),P(3)-bis(5'-adenosyl) triphosphate(4-).
Diadenosine triphosphate is a metabolite found in or produced by Escherichia coli (strain K12, MG1655).
This compound is a natural product found in Bos taurus with data available.
生物活性
Diadenosine triphosphate (Ap3A) is a member of the diadenosine polyphosphate family, which includes other compounds like diadenosine tetraphosphate (Ap4A). Ap3A has garnered attention due to its diverse biological activities and potential roles in cellular signaling, metabolism, and stress responses. This article delves into the biological activity of Ap3A, summarizing key findings from various studies, including case studies and research data.
Chemical Structure and Properties
This compound consists of two adenosine molecules linked by a triphosphate chain. Its chemical structure allows it to interact with various proteins and enzymes, influencing numerous cellular processes. The unique conformation of Ap3A enables it to bind to specific receptors and modulate physiological responses.
Biological Functions
1. Role in Platelet Aggregation:
Ap3A is known to play a significant role in modulating platelet aggregation. Studies have shown that when added to platelet-rich plasma, Ap3A can induce aggregation at concentrations ranging from 10-20 µM. This effect is enhanced when combined with other stimuli such as platelet-activating factor (PAF), while Ap4A acts as an antagonist, disaggregating platelets .
2. Stress Response and Alarmone Function:
Research indicates that Ap3A acts as an alarmone during cellular stress. Its concentration increases significantly under stress conditions, suggesting a role in adaptive responses. For example, in yeast models, increased levels of Ap4A were observed during stress, implicating a similar function for Ap3A . This response is critical for maintaining cellular homeostasis and regulating metabolic pathways.
3. Interaction with Proteins:
Chemical proteomic profiling has identified numerous protein interactors of Ap3A, highlighting its involvement in various fundamental cellular processes including metabolism and gene expression . These interactions suggest that Ap3A may act as a signaling molecule that influences protein activity and cellular responses.
Case Studies
- Human Platelets: A study demonstrated that Ap3A is stored in significant quantities within human platelets and released upon thrombin-induced aggregation. The presence of Ap3A prolongs the aggregatory effect of other stimuli, indicating its role as a long-lived signaling molecule .
- Filarial Parasites: In research on Brugia malayi (a filarial parasite), significant amounts of Ap3A were detected using mass spectrometry. This finding underscores the importance of diadenosine oligophosphates in eukaryotic pathogens and their potential roles in pathophysiological processes .
Data Table: Biological Activities of this compound
The mechanisms through which Ap3A exerts its effects are multifaceted:
- Receptor Interaction: Ap3A interacts with purinergic receptors on cell surfaces, influencing signal transduction pathways.
- Metabolic Regulation: It modulates metabolic pathways by interacting with enzymes involved in nucleotide metabolism.
- Gene Expression Modulation: By affecting transcription factors and other regulatory proteins, Ap3A can influence gene expression patterns under varying physiological conditions.
特性
IUPAC Name |
bis[[(2R,3S,4R,5R)-5-(6-aminopurin-9-yl)-3,4-dihydroxyoxolan-2-yl]methoxy-hydroxyphosphoryl] hydrogen phosphate | |
---|---|---|
Source | PubChem | |
URL | https://pubchem.ncbi.nlm.nih.gov | |
Description | Data deposited in or computed by PubChem | |
InChI |
InChI=1S/C20H27N10O16P3/c21-15-9-17(25-3-23-15)29(5-27-9)19-13(33)11(31)7(43-19)1-41-47(35,36)45-49(39,40)46-48(37,38)42-2-8-12(32)14(34)20(44-8)30-6-28-10-16(22)24-4-26-18(10)30/h3-8,11-14,19-20,31-34H,1-2H2,(H,35,36)(H,37,38)(H,39,40)(H2,21,23,25)(H2,22,24,26)/t7-,8-,11-,12-,13-,14-,19-,20-/m1/s1 | |
Source | PubChem | |
URL | https://pubchem.ncbi.nlm.nih.gov | |
Description | Data deposited in or computed by PubChem | |
InChI Key |
QCICUPZZLIQAPA-XPWFQUROSA-N | |
Source | PubChem | |
URL | https://pubchem.ncbi.nlm.nih.gov | |
Description | Data deposited in or computed by PubChem | |
Canonical SMILES |
C1=NC(=C2C(=N1)N(C=N2)C3C(C(C(O3)COP(=O)(O)OP(=O)(O)OP(=O)(O)OCC4C(C(C(O4)N5C=NC6=C(N=CN=C65)N)O)O)O)O)N | |
Source | PubChem | |
URL | https://pubchem.ncbi.nlm.nih.gov | |
Description | Data deposited in or computed by PubChem | |
Isomeric SMILES |
C1=NC(=C2C(=N1)N(C=N2)[C@H]3[C@@H]([C@@H]([C@H](O3)COP(=O)(O)OP(=O)(O)OP(=O)(O)OC[C@@H]4[C@H]([C@H]([C@@H](O4)N5C=NC6=C(N=CN=C65)N)O)O)O)O)N | |
Source | PubChem | |
URL | https://pubchem.ncbi.nlm.nih.gov | |
Description | Data deposited in or computed by PubChem | |
Molecular Formula |
C20H27N10O16P3 | |
Source | PubChem | |
URL | https://pubchem.ncbi.nlm.nih.gov | |
Description | Data deposited in or computed by PubChem | |
Molecular Weight |
756.4 g/mol | |
Source | PubChem | |
URL | https://pubchem.ncbi.nlm.nih.gov | |
Description | Data deposited in or computed by PubChem | |
CAS No. |
5959-90-0 | |
Record name | Ap3A | |
Source | CAS Common Chemistry | |
URL | https://commonchemistry.cas.org/detail?cas_rn=5959-90-0 | |
Description | CAS Common Chemistry is an open community resource for accessing chemical information. Nearly 500,000 chemical substances from CAS REGISTRY cover areas of community interest, including common and frequently regulated chemicals, and those relevant to high school and undergraduate chemistry classes. This chemical information, curated by our expert scientists, is provided in alignment with our mission as a division of the American Chemical Society. | |
Explanation | The data from CAS Common Chemistry is provided under a CC-BY-NC 4.0 license, unless otherwise stated. | |
Record name | Adenosine 5'-triphosphate 5'-adenosine | |
Source | ChemIDplus | |
URL | https://pubchem.ncbi.nlm.nih.gov/substance/?source=chemidplus&sourceid=0005959900 | |
Description | ChemIDplus is a free, web search system that provides access to the structure and nomenclature authority files used for the identification of chemical substances cited in National Library of Medicine (NLM) databases, including the TOXNET system. | |
Retrosynthesis Analysis
AI-Powered Synthesis Planning: Our tool employs the Template_relevance Pistachio, Template_relevance Bkms_metabolic, Template_relevance Pistachio_ringbreaker, Template_relevance Reaxys, Template_relevance Reaxys_biocatalysis model, leveraging a vast database of chemical reactions to predict feasible synthetic routes.
One-Step Synthesis Focus: Specifically designed for one-step synthesis, it provides concise and direct routes for your target compounds, streamlining the synthesis process.
Accurate Predictions: Utilizing the extensive PISTACHIO, BKMS_METABOLIC, PISTACHIO_RINGBREAKER, REAXYS, REAXYS_BIOCATALYSIS database, our tool offers high-accuracy predictions, reflecting the latest in chemical research and data.
Strategy Settings
Precursor scoring | Relevance Heuristic |
---|---|
Min. plausibility | 0.01 |
Model | Template_relevance |
Template Set | Pistachio/Bkms_metabolic/Pistachio_ringbreaker/Reaxys/Reaxys_biocatalysis |
Top-N result to add to graph | 6 |
Feasible Synthetic Routes
Q1: How does Ap3A exert its biological effects?
A1: Ap3A interacts with various cellular targets, including enzymes and receptors. Notably, it is hydrolyzed by the tumor suppressor protein Fhit (fragile histidine triad) to produce adenosine monophosphate (AMP) and adenosine diphosphate (ADP) []. This hydrolysis is believed to be crucial for the tumor-suppressive function of Fhit []. Additionally, Ap3A can interact with purinergic receptors, influencing cellular processes like vasodilation and platelet aggregation [, , ].
Q2: What are the downstream effects of Ap3A hydrolysis by Fhit?
A2: While the exact mechanisms are still under investigation, the Fhit-Ap3A complex has been shown to impede translation, leading to reduced cell viability []. This suggests a role for Ap3A in regulating cellular proliferation and potentially contributing to Fhit's tumor-suppressive activity.
Q3: What is the molecular formula and weight of Ap3A?
A3: The molecular formula of Ap3A is C20H25N10O13P3. Its molecular weight is 674.4 g/mol.
Q4: Is there any spectroscopic data available for Ap3A?
A4: While specific spectroscopic data wasn't detailed in the provided research, techniques like mass spectrometry have been used to identify Ap3A and its degradation products [, ]. Other common techniques for nucleotide characterization include NMR spectroscopy and UV-Vis spectroscopy.
Q5: How stable is Ap3A under various conditions?
A5: Ap3A degradation rates vary depending on the environment. For instance, Ap3A hydrolysis is significantly slower in citrated plasma compared to heparinized plasma due to the requirement of divalent metal ions for the hydrolase enzyme [].
Q6: Are there enzymes besides Fhit that can hydrolyze Ap3A?
A6: Yes, Ap3A can be hydrolyzed by other enzymes, including ectonucleotide pyrophosphatase/phosphodiesterase-1 (ENPP1) []. This enzyme, found on the surface of cells like intestinal epithelia, breaks down Ap3A to ADP and AMP, contributing to extracellular adenosine signaling [].
Q7: Can Ap3A be synthesized enzymatically?
A7: Yes, a mutated form of Fhit (H96G-Fhit) can catalyze the synthesis of Ap3A and other dinucleoside polyphosphates from nucleoside-5'-phosphimidazolides and nucleoside di- or triphosphates []. This highlights the potential for engineering enzymes for the production of Ap3A and its analogs.
Q8: Have there been computational studies on Ap3A?
A8: While the provided research doesn't delve into detailed computational studies, it highlights the use of X-ray crystallography to analyze Ap3A binding to proteins like Fhit and RNase A [, ]. These structural insights can be used in computational models to further understand Ap3A interactions and design potential inhibitors.
Q9: How does modifying the structure of Ap3A impact its activity?
A9: Structural modifications can significantly alter Ap3A's biological activity. For example, adding a 5'-pyrophosphate-linked extension to Ap3A influences its binding to Ribonuclease A, highlighting the importance of specific structural features for target interaction [].
Q10: What are the implications of Ap3A's presence in different tissues?
A10: The presence of Ap3A in various tissues, including platelets, cardiac tissue, and placenta, suggests diverse physiological roles [, , ]. For instance, its release from platelet granules during aggregation implicates Ap3A in hemostasis and potentially in the pathophysiology of cardiovascular diseases [, , ].
Q11: Are there any known inhibitors of Fhit's Ap3A hydrolase activity?
A11: Yes, small molecule inhibitors of Fhit have been identified through high-throughput screening efforts []. These inhibitors show promise as tools for studying Fhit's function and its role in cancer development.
Q12: What is the historical context of Ap3A research?
A12: Ap3A was first identified in the 1960s, and research has continued to unravel its diverse biological functions []. Early studies focused on its presence in platelets and its potential role in platelet aggregation []. Subsequent research revealed its involvement in cellular processes like signal transduction, DNA replication, and apoptosis, highlighting its significance in cell biology [].
試験管内研究製品の免責事項と情報
BenchChemで提示されるすべての記事および製品情報は、情報提供を目的としています。BenchChemで購入可能な製品は、生体外研究のために特別に設計されています。生体外研究は、ラテン語の "in glass" に由来し、生物体の外で行われる実験を指します。これらの製品は医薬品または薬として分類されておらず、FDAから任何の医療状態、病気、または疾患の予防、治療、または治癒のために承認されていません。これらの製品を人間または動物に体内に導入する形態は、法律により厳格に禁止されています。これらのガイドラインに従うことは、研究と実験において法的および倫理的な基準の遵守を確実にするために重要です。