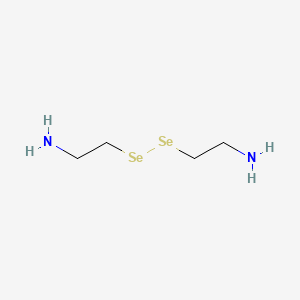
Selenocystamine
説明
Selenocystamine is a compound that contains selenium and is commonly used in scientific research applications. It is a derivative of cystamine, which is a compound that has been studied for its potential therapeutic effects on various diseases. The addition of selenium to cystamine has been shown to enhance its biological activity and make it a more effective therapeutic agent.
科学的研究の応用
Photodynamic Therapy (PDT) for Cervical Carcinoma Cells
- Application : Researchers have developed ROS-sensitive nanophotosensitizers by conjugating chlorin e6 (Ce6) with succinyl β-cyclodextrin via selenocystamine linkages. These nanophotosensitizers specifically target cervical cancer cells through folate-receptor-mediated delivery .
Ce6 Tetramer Formation for Enhanced PDT
- Application : Ce6 was conjugated with tetra acid (TA) via selenocystamine linkages to create Ce6 tetramers. These tetramers may enhance PDT efficacy .
ROS-Sensitive Nanoparticles for Drug Delivery
- Application : Selenocystamine linkages were introduced between PLGA (poly(lactic-co-glycolic acid)) and TA (3-[3-(2-carboxyethoxy)-2,2-bis(2-carboxyethoxymethyl)propoxy]propanoic acid) to create ROS-sensitive nanoparticles. These nanoparticles can release drugs in response to ROS levels .
将来の方向性
: Tuo, Q.-Z., Masaldan, S., Southon, A., Mawal, C., Ayton, S., Bush, A. I., Lei, P., & Belaidi, A. A. (2021). Characterization of Selenium Compounds for Anti-ferroptotic Activity in Neuronal Cells and After Cerebral Ischemia–Reperfusion Injury. Neurotherapeutics, 18(9), 2682–2691. Read more
: Selenium is an essential element in humans and is present in various inorganic and organic forms. Selenomethionine is more bioavailable than inorganic forms such as selenite and selenate. Animal studies suggest preferential retention of selenium in the brain under selenium-deficient conditions, and lower selenium levels are associated with cognitive decline and oxidative stress in the elderly. Source
: Beld, J., Woycechowsky, K. J., Hilvert, D., & Saven, J. G. (2010). Mechanism of Selenocystamine-induced Refolding of Aggregated Firefly Luciferase. Journal of Molecular Biology, 397(4), 1077–1088. Read more
作用機序
Target of Action
Selenocystamine is a naturally occurring amino acid found in both eukaryotic and prokaryotic organisms . It is present in transfer RNAs (tRNAs) and in the catalytic site of some enzymes . The genes for glutathione peroxidase and formate dehydrogenase contain the TGA codon, which codes for this amino acid .
Mode of Action
Selenocystamine is capable of catalyzing the formation of disulfide bonds . This ability to oxidize proteins that require disulfide bonds into a stable conformation is a crucial aspect of its mode of action .
Biochemical Pathways
Selenocystamine plays a significant role in the formation of disulfide bonds in proteins, which is a critical step in protein folding and stability . It is involved in the antioxidant pathways, facilitating the oxidation of glutathione .
Pharmacokinetics
For instance, the bioavailability of Selenocystamine can be decreased when combined with Eltrombopag .
Result of Action
The primary result of Selenocystamine’s action is the increased accumulation of proteins containing disulfide bonds . This suggests that a rate-limiting step in protein accumulation is the formation of disulfide bonds in the native structure of recombinant proteins .
Action Environment
The action of Selenocystamine can be influenced by various environmental factors. For instance, in the context of chloroplast protein accumulation, Selenocystamine’s ability to increase the accumulation of proteins containing disulfide bonds suggests that the formation of these bonds is a rate-limiting step .
特性
IUPAC Name |
2-(2-aminoethyldiselanyl)ethanamine | |
---|---|---|
Source | PubChem | |
URL | https://pubchem.ncbi.nlm.nih.gov | |
Description | Data deposited in or computed by PubChem | |
InChI |
InChI=1S/C4H12N2Se2/c5-1-3-7-8-4-2-6/h1-6H2 | |
Source | PubChem | |
URL | https://pubchem.ncbi.nlm.nih.gov | |
Description | Data deposited in or computed by PubChem | |
InChI Key |
QNGIKJLVQNCRRC-UHFFFAOYSA-N | |
Source | PubChem | |
URL | https://pubchem.ncbi.nlm.nih.gov | |
Description | Data deposited in or computed by PubChem | |
Canonical SMILES |
C(C[Se][Se]CCN)N | |
Source | PubChem | |
URL | https://pubchem.ncbi.nlm.nih.gov | |
Description | Data deposited in or computed by PubChem | |
Molecular Formula |
C4H12N2Se2 | |
Source | PubChem | |
URL | https://pubchem.ncbi.nlm.nih.gov | |
Description | Data deposited in or computed by PubChem | |
DSSTOX Substance ID |
DTXSID10181470 | |
Record name | Selenocystamine | |
Source | EPA DSSTox | |
URL | https://comptox.epa.gov/dashboard/DTXSID10181470 | |
Description | DSSTox provides a high quality public chemistry resource for supporting improved predictive toxicology. | |
Molecular Weight |
246.09 g/mol | |
Source | PubChem | |
URL | https://pubchem.ncbi.nlm.nih.gov | |
Description | Data deposited in or computed by PubChem | |
CAS RN |
2697-61-2 | |
Record name | Selenocystamine | |
Source | ChemIDplus | |
URL | https://pubchem.ncbi.nlm.nih.gov/substance/?source=chemidplus&sourceid=0002697612 | |
Description | ChemIDplus is a free, web search system that provides access to the structure and nomenclature authority files used for the identification of chemical substances cited in National Library of Medicine (NLM) databases, including the TOXNET system. | |
Record name | Selenocystamine | |
Source | EPA DSSTox | |
URL | https://comptox.epa.gov/dashboard/DTXSID10181470 | |
Description | DSSTox provides a high quality public chemistry resource for supporting improved predictive toxicology. | |
Retrosynthesis Analysis
AI-Powered Synthesis Planning: Our tool employs the Template_relevance Pistachio, Template_relevance Bkms_metabolic, Template_relevance Pistachio_ringbreaker, Template_relevance Reaxys, Template_relevance Reaxys_biocatalysis model, leveraging a vast database of chemical reactions to predict feasible synthetic routes.
One-Step Synthesis Focus: Specifically designed for one-step synthesis, it provides concise and direct routes for your target compounds, streamlining the synthesis process.
Accurate Predictions: Utilizing the extensive PISTACHIO, BKMS_METABOLIC, PISTACHIO_RINGBREAKER, REAXYS, REAXYS_BIOCATALYSIS database, our tool offers high-accuracy predictions, reflecting the latest in chemical research and data.
Strategy Settings
Precursor scoring | Relevance Heuristic |
---|---|
Min. plausibility | 0.01 |
Model | Template_relevance |
Template Set | Pistachio/Bkms_metabolic/Pistachio_ringbreaker/Reaxys/Reaxys_biocatalysis |
Top-N result to add to graph | 6 |
Feasible Synthetic Routes
Q & A
Q1: How does Selenocystamine inhibit influenza virus replication?
A1: Selenocystamine inhibits the particle-associated RNA-dependent RNA polymerase activity of influenza A and B viruses. [] This enzyme is essential for viral replication. []
Q2: Can Selenocystamine's inhibitory effect on influenza virus be reversed?
A2: Yes, the inhibitory activity of Selenocystamine can be completely reversed by adding β-mercaptoethanol and partially reversed through dialysis. [] This suggests a reversible interaction with the target.
Q3: How does Selenocystamine interact with G protein βγ subunits?
A4: Selenocystamine inhibits G protein βγ subunits through a reversible redox mechanism, primarily targeting the GβCys204 residue. [, ] It forms a selenylsulfide bridge with this cysteine residue, disrupting the protein-protein interactions of Gβγ. []
Q4: Can the interaction between Selenocystamine and G protein βγ subunits be reversed?
A5: Yes, the inhibitory effect of Selenocystamine on G protein βγ subunits can be reversed by adding dithiothreitol (DTT), indicating a reversible redox reaction. []
Q5: What is the molecular formula and weight of Selenocystamine?
A5: Selenocystamine has a molecular formula of C4H12N2Se2 and a molecular weight of 214.11 g/mol.
Q6: Is Selenocystamine stable in alkaline conditions?
A7: In the presence of cupric ions and alkaline conditions, Selenocystamine undergoes autoxidation and transforms into selenohypotaurine. []
Q7: Does Selenocystamine possess glutathione peroxidase-like activity?
A8: While Selenocystamine itself doesn't exhibit direct glutathione peroxidase-like activity, conjugates like Heparin-Selenocystamine, designed to mimic heparin-selenoprotein P complex, do exhibit this activity. []
Q8: How does Selenocystamine contribute to the antioxidant activity of Heparin-Selenocystamine conjugate?
A9: In Heparin-Selenocystamine conjugate, Selenocystamine's diselenide bond is cleaved during the conjugation process, leaving a stable selenol group. [] This selenol group is responsible for the conjugate's glutathione peroxidase-like activity and scavenging activity against hydrogen peroxide, tert-butyl hydroperoxide, cumene hydroperoxide, DPPH radicals, and superoxide anions. []
Q9: Can Selenocystamine catalyze the decomposition of S-nitrosothiols?
A10: Yes, Selenocystamine can catalyze the decomposition of S-nitrosothiols like S-nitrosoglutathione and S-nitroso-N-acetyl-D, L-penicillamine in the presence of thiols, releasing nitric oxide. []
Q10: How does the replacement of sulfur with selenium in cystamine affect its interaction with G protein βγ subunits?
A11: Replacing sulfur with selenium in cystamine to form Selenocystamine significantly enhances its inhibitory potency towards G protein βγ subunits, demonstrating the importance of selenium for its activity. []
Q11: How can Selenocystamine be used in targeted drug delivery systems?
A14: Selenocystamine can be incorporated into nanoparticles designed for targeted drug delivery. For example, it has been used to create ROS-sensitive nanoparticles for ciprofloxacin delivery to treat urinary tract infections. []
Q12: Can Selenocystamine be used for targeted photodynamic therapy?
A15: Yes, Selenocystamine has been used to develop folate receptor-targeted nanophotosensitizers for photodynamic therapy. These nanophotosensitizers, composed of folic acid, poly(ethylene glycol), and chlorin e6 tetramer linked by Selenocystamine, demonstrate ROS sensitivity and enhanced drug delivery to folate receptor-overexpressing cancer cells. []
Q13: How is Selenocystamine typically determined in biological samples?
A16: Selenocystamine can be determined in biological samples using techniques like high performance liquid chromatography (HPLC) coupled with inductively coupled plasma mass spectrometry (ICP-MS). [] This allows for the separation and sensitive detection of Selenocystamine.
Q14: What specific analytical challenges are associated with Selenocystamine determination?
A17: One challenge is the low recovery of Selenocystamine from samples like herring gull eggs using traditional hot water extraction methods, which yielded only 8% recovery. [] This highlights the need for optimized extraction procedures.
Q15: Can Selenocystamine be selectively preconcentrated from complex matrices?
A18: Yes, living Pseudomonas putida cells can selectively preconcentrate Selenocystamine from water samples containing other selenium species. [] This approach improves the sensitivity and selectivity of Selenocystamine determination by slurry sampling electrothermal atomic absorption spectrometry.
Q16: How does the presence of other selenium species affect Selenocystamine determination?
A19: The presence of other selenium species like selenoethionine, Se(IV), selenomethionine, selenourea, selenocystine, and Se(VI) can interfere with Selenocystamine determination. [] This highlights the importance of selective preconcentration or separation techniques for accurate speciation analysis.
Q17: What is known about the environmental fate and degradation of Selenocystamine?
A20: While specific information on the environmental fate and degradation of Selenocystamine is limited within the provided research, its presence as a selenocystamine fraction in herring gull eggs from the North Sea suggests potential bioaccumulation and food web transfer. [, ] Further research is needed to understand its environmental behavior fully.
試験管内研究製品の免責事項と情報
BenchChemで提示されるすべての記事および製品情報は、情報提供を目的としています。BenchChemで購入可能な製品は、生体外研究のために特別に設計されています。生体外研究は、ラテン語の "in glass" に由来し、生物体の外で行われる実験を指します。これらの製品は医薬品または薬として分類されておらず、FDAから任何の医療状態、病気、または疾患の予防、治療、または治癒のために承認されていません。これらの製品を人間または動物に体内に導入する形態は、法律により厳格に禁止されています。これらのガイドラインに従うことは、研究と実験において法的および倫理的な基準の遵守を確実にするために重要です。