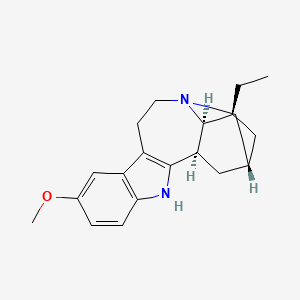
Ibogaine
概要
説明
Ibogaine is a naturally occurring psychoactive indole alkaloid found in several plant species, most notably in the root bark of the African shrub Tabernanthe iboga. It has been traditionally used in West African spiritual ceremonies and rituals. This compound is known for its potential therapeutic effects, particularly in the treatment of substance use disorders, due to its ability to reduce withdrawal symptoms and cravings .
準備方法
Synthetic Routes and Reaction Conditions: Ibogaine can be synthesized through several methods. One common approach involves the semi-synthesis from voacangine, another plant alkaloid. The total synthesis of this compound was first described in 1956. The process typically involves multiple steps, including the formation of key intermediates and the use of specific reagents and catalysts to achieve the desired chemical transformations .
Industrial Production Methods: Industrial production of this compound often relies on the extraction from the root bark of Tabernanthe iboga. The extraction process involves the use of solvents to isolate the alkaloid, followed by purification steps to obtain this compound in its pure form. Semi-synthetic methods using voacangine as a precursor are also employed in industrial settings .
化学反応の分析
Types of Reactions: Ibogaine undergoes various chemical reactions, including oxidation, reduction, and substitution reactions. These reactions are essential for modifying the structure of this compound and exploring its derivatives for potential therapeutic applications .
Common Reagents and Conditions:
Oxidation: Common oxidizing agents such as potassium permanganate or chromium trioxide can be used to oxidize this compound, leading to the formation of different oxidation products.
Reduction: Reducing agents like lithium aluminum hydride or sodium borohydride are employed to reduce this compound, resulting in the formation of reduced derivatives.
Substitution: Substitution reactions often involve the use of halogens or other electrophiles to introduce new functional groups into the this compound molecule
Major Products: The major products formed from these reactions include various this compound derivatives, which are studied for their potential pharmacological properties. These derivatives can exhibit different levels of psychoactivity and therapeutic potential .
科学的研究の応用
Treatment of Opioid Use Disorder
Numerous studies have investigated ibogaine's efficacy in treating opioid use disorder (OUD). A systematic review identified 24 studies involving 705 participants, indicating that this compound can significantly reduce withdrawal symptoms and cravings associated with opioids .
- Key Findings:
- A study involving 50 participants undergoing detoxification reported a significant reduction in withdrawal symptoms and cravings within 48 hours post-treatment .
- An observational study in New Zealand showed that a single this compound treatment led to sustained reductions in opioid use over a 12-month follow-up period .
Study | Sample Size | Duration | Key Outcomes |
---|---|---|---|
Noller et al. (2018) | 14 | 12 months | Significant reductions in Addiction Severity Index scores (p=0.002) and Beck Depression Inventory scores (p<0.001) |
Malcolm et al. (2018) | 50 | 1 week | 78% of participants showed no objective signs of withdrawal at 48 hours post-treatment |
Healthline Observational Studies (2017) | Varied | Up to 8 months | Median abstinence duration of 5.5 months for single treatment; 8.4 months for multiple treatments |
Safety and Side Effects
While this compound shows promise, it is not without risks. Clinical trials have reported severe adverse effects, including cardiac arrhythmias and fatalities associated with its use .
- Adverse Effects Noted:
- Ataxia (impaired coordination)
- Nausea
- Cardiac complications leading to fatalities in some cases
Case Studies
Several case studies have documented individual experiences with this compound treatment:
- Case Study A: A participant with severe opioid dependence underwent a single this compound treatment and reported significant reductions in cravings and withdrawal symptoms lasting for several months post-treatment.
- Case Study B: Another individual experienced immediate alleviation of withdrawal symptoms but faced complications requiring medical intervention due to cardiovascular issues.
作用機序
Ibogaine exerts its effects through a complex mechanism involving multiple molecular targets and pathways:
Neurotransmitter Systems: this compound interacts with serotonin, dopamine, and opioid receptors, modulating their activity and influencing neurotransmitter release and reuptake.
Neuroplasticity: this compound promotes the expression of neurotrophic factors such as brain-derived neurotrophic factor (BDNF) and glial cell line-derived neurotrophic factor (GDNF), which play a role in neuronal growth and survival.
Opioid Receptors: this compound acts as a weak antagonist at mu opioid receptors, contributing to its anti-craving and detoxification effects
類似化合物との比較
Ibogaine is part of a family of structurally similar alkaloids known as iboga alkaloids. Some of the similar compounds include:
Northis compound: A metabolite of this compound with similar anti-addictive properties but reduced psychoactivity.
18-Methoxycoronaridine (18-MC): A synthetic derivative of this compound designed to lack psychedelic effects while retaining anti-addictive properties.
Tabernanthalog (TBG): A non-hallucinogenic analogue of this compound developed to treat addiction and depression with fewer side effects .
This compound’s uniqueness lies in its broad spectrum of activity across multiple neurotransmitter systems and its potential to induce long-lasting changes in brain function, making it a promising candidate for treating various neurological and psychiatric disorders.
生物活性
Ibogaine is a naturally occurring psychoactive compound derived from the root bark of the African shrub Tabernanthe iboga. It has garnered attention for its potential therapeutic effects, particularly in treating substance use disorders (SUDs), including opioid addiction. This article explores the biological activity of this compound, focusing on its pharmacodynamics, case studies, and research findings.
This compound exerts its effects through multiple mechanisms, primarily involving the modulation of neurotransmitter systems. It interacts with various receptors, including:
- Serotonin Receptors (5-HT2A) : this compound's hallucinogenic effects are partly mediated by its action on serotonin receptors, particularly 5-HT2A.
- Opioid Receptors : this compound has been shown to interact with mu (MOR), delta (DOR), and kappa (KOR) opioid receptors, which may contribute to its analgesic and anti-addictive properties.
- Norepinephrine and Dopamine Systems : The compound influences norepinephrine and dopamine pathways, which are crucial in mood regulation and reward processing.
Biological Activity Overview
Research indicates that this compound's biological activity can lead to various physiological effects. Below is a summary of key findings:
Case Studies
Several case studies highlight this compound's therapeutic potential:
-
Neuropathic Pain Management :
- A 53-year-old male with severe neuropathic pain reported a 72.2% reduction in pain after a single this compound treatment, achieving a complete reduction in pain measures after a saturation protocol. This suggests that this compound could be an effective treatment for chronic pain conditions resistant to conventional therapies .
- Opioid Dependence Treatment :
- Long-term Efficacy :
Antioxidant Activity and Oxidative Stress
Research has demonstrated that this compound influences cellular redox states. Low concentrations can stimulate contractile activity in uterine tissues, while higher doses inhibit contractions by altering antioxidant enzyme activities, indicating a dose-dependent effect on oxidative stress levels .
Cardiotoxicity Concerns
Studies have shown that this compound can lead to cardiotoxic necrosis in rats at higher doses, which raises significant safety concerns for its clinical use . The relationship between this compound dosage and cardiac health necessitates further investigation to understand the mechanisms underlying these adverse effects.
特性
CAS番号 |
83-74-9 |
---|---|
分子式 |
C20H26N2O |
分子量 |
310.4 g/mol |
IUPAC名 |
(1R,15S)-17-ethyl-7-methoxy-3,13-diazapentacyclo[13.3.1.02,10.04,9.013,18]nonadeca-2(10),4(9),5,7-tetraene |
InChI |
InChI=1S/C20H26N2O/c1-3-13-8-12-9-17-19-15(6-7-22(11-12)20(13)17)16-10-14(23-2)4-5-18(16)21-19/h4-5,10,12-13,17,20-21H,3,6-9,11H2,1-2H3/t12-,13?,17-,20?/m0/s1 |
InChIキー |
HSIBGVUMFOSJPD-WBPAKMTCSA-N |
SMILES |
CCC1CC2CC3C1N(C2)CCC4=C3NC5=C4C=C(C=C5)OC |
異性体SMILES |
CCC1C[C@H]2C[C@@H]3C1N(C2)CCC4=C3NC5=C4C=C(C=C5)OC |
正規SMILES |
CCC1CC2CC3C1N(C2)CCC4=C3NC5=C4C=C(C=C5)OC |
melting_point |
148 °C Crystals from ethyl acetate. mp: 132 °C /Ibopamine hydrochloride/ |
Key on ui other cas no. |
83-74-9 |
ピクトグラム |
Irritant |
賞味期限 |
Stable under recommended storage conditions. /Ibogaine hydrochloride/ |
溶解性 |
Soluble in chloroform |
同義語 |
12 Methoxyibogamine 12-Methoxyibogamine Endabuse Ibogaine NIH 10567 NIH-10567 NIH10567 |
蒸気圧 |
3.03X10-8 mm Hg at 25 °C (est) |
製品の起源 |
United States |
Retrosynthesis Analysis
AI-Powered Synthesis Planning: Our tool employs the Template_relevance Pistachio, Template_relevance Bkms_metabolic, Template_relevance Pistachio_ringbreaker, Template_relevance Reaxys, Template_relevance Reaxys_biocatalysis model, leveraging a vast database of chemical reactions to predict feasible synthetic routes.
One-Step Synthesis Focus: Specifically designed for one-step synthesis, it provides concise and direct routes for your target compounds, streamlining the synthesis process.
Accurate Predictions: Utilizing the extensive PISTACHIO, BKMS_METABOLIC, PISTACHIO_RINGBREAKER, REAXYS, REAXYS_BIOCATALYSIS database, our tool offers high-accuracy predictions, reflecting the latest in chemical research and data.
Strategy Settings
Precursor scoring | Relevance Heuristic |
---|---|
Min. plausibility | 0.01 |
Model | Template_relevance |
Template Set | Pistachio/Bkms_metabolic/Pistachio_ringbreaker/Reaxys/Reaxys_biocatalysis |
Top-N result to add to graph | 6 |
Feasible Synthetic Routes
Q1: How does ibogaine exert its effects within the body?
A1: this compound interacts with various neurotransmitter systems, exhibiting micromolar affinity for N-methyl-D-aspartate (NMDA), κ-opioid, μ-opioid, and sigma-2 receptors []. It also interacts with acetylcholine, serotonin, and dopamine systems, influencing the expression of proteins like substance P, brain-derived neurotrophic factor (BDNF), c-fos, and egr-1 []. This compound's primary metabolite, northis compound, also demonstrates biological activity, significantly contributing to this compound's overall pharmacological profile [].
Q2: What are the key differences in the pharmacological profiles of this compound and northis compound?
A2: While both this compound and northis compound exhibit anti-addictive properties in animal models, they differ in potency and side-effect profile. Notably, northis compound is a more potent indirect serotonin agonist than this compound, demonstrating a 10-fold higher potency in increasing extracellular serotonin levels in the nucleus accumbens of rats []. Additionally, northis compound appears to have a reduced propensity to induce adverse effects like tremors compared to this compound, suggesting it might be a safer alternative for medication development [].
Q3: What role does glial cell line-derived neurotrophic factor (GDNF) play in this compound's actions against alcohol consumption?
A4: Research suggests that GDNF in the ventral tegmental area (VTA) is crucial in mediating this compound's effects on reducing alcohol consumption []. this compound has been shown to increase GDNF expression in the VTA, and this increase is associated with a reduction in ethanol self-administration in rodents [, ]. The effect of this compound on ethanol consumption can be mimicked by injecting GDNF directly into the VTA and can be attenuated by injecting anti-GDNF antibodies, further supporting the critical role of GDNF in this compound's actions [].
Q4: How is this compound metabolized in the body?
A5: this compound is primarily metabolized by the cytochrome P450 enzyme CYP2D6 to its main metabolite, northis compound [, , ]. Northis compound exhibits clinically relevant concentrations for an extended period, even after this compound is cleared from the system []. This prolonged presence of northis compound is likely due to its slow release from fat tissue, where both this compound and northis compound are sequestered [].
Q5: What is the significance of CYP2D6 polymorphisms in this compound metabolism?
A6: Genetic variations in the CYP2D6 enzyme can significantly influence the pharmacokinetic profiles of both this compound and northis compound [, ]. Patients identified as CYP2D6 poor metabolizers exhibit a 3-fold higher blood concentration of this compound and a 4-fold lower concentration of northis compound compared to extensive metabolizers []. This suggests that individuals with different CYP2D6 metabolic phenotypes may exhibit varied sensitivity to this compound and northis compound, potentially influencing therapeutic outcomes and safety profiles [].
Q6: What are the implications of this compound and northis compound sequestration in fat tissue?
A7: Both this compound and its active metabolite, northis compound, are sequestered in fat tissue [, ]. This sequestration contributes to their complex pharmacokinetic profiles, characterized by a long elimination half-life and prolonged presence in the body even after a single dose [, ]. The slow release of this compound and northis compound from fat stores could contribute to the long-lasting effects observed after a single administration [].
Q7: Are there alternative compounds being explored that might offer a safer profile than this compound?
A11: The development of safer analogs of this compound with reduced toxicity is an active area of research []. One such compound, 18-methoxycoronaridine (18-MC), has shown promise in preclinical studies. 18-MC, a synthetic derivative of this compound, exhibits similar anti-addictive properties in rodent models without some of this compound's adverse side effects, such as tremors and cerebellar damage []. Its lower affinity for NMDA and sigma-2 receptors, compared to this compound, is thought to contribute to its improved safety profile [].
Q8: What are the critical areas for future research on this compound?
A12: Future research should prioritize well-controlled clinical trials to assess the long-term safety and efficacy of this compound in treating substance use disorders [, , ]. Exploring personalized medicine approaches based on CYP2D6 genotyping could potentially optimize dosing and minimize adverse effects []. Further investigation into the mechanisms of action, particularly the role of different receptors and signaling pathways, is crucial for understanding this compound's complex pharmacology and developing safer and more effective therapies [].
試験管内研究製品の免責事項と情報
BenchChemで提示されるすべての記事および製品情報は、情報提供を目的としています。BenchChemで購入可能な製品は、生体外研究のために特別に設計されています。生体外研究は、ラテン語の "in glass" に由来し、生物体の外で行われる実験を指します。これらの製品は医薬品または薬として分類されておらず、FDAから任何の医療状態、病気、または疾患の予防、治療、または治癒のために承認されていません。これらの製品を人間または動物に体内に導入する形態は、法律により厳格に禁止されています。これらのガイドラインに従うことは、研究と実験において法的および倫理的な基準の遵守を確実にするために重要です。