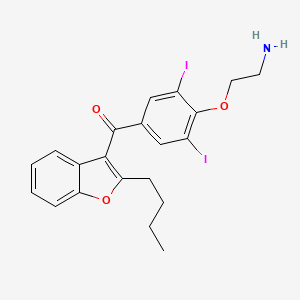
Di-N-desethylamiodarone
概要
説明
Di-N-desethylamiodarone is a metabolite of the widely used antiarrhythmic drug amiodarone. Amiodarone is known for its efficacy in treating various cardiac arrhythmias, including atrial fibrillation and ventricular tachycardia. This compound is formed through the metabolic process involving the removal of ethyl groups from amiodarone. This compound retains some of the pharmacological properties of its parent compound and has been the subject of various scientific studies due to its unique characteristics .
準備方法
Synthetic Routes and Reaction Conditions
Di-N-desethylamiodarone is synthesized through the N-demethylation of amiodarone. This process is catalyzed by cytochrome P450 enzymes, primarily CYP3A4. The reaction involves the removal of ethyl groups from the nitrogen atoms in the amiodarone molecule .
Industrial Production Methods
In industrial settings, the production of this compound involves the use of high-performance liquid chromatography (HPLC) for the separation and purification of the compound. The process typically includes the extraction of amiodarone from biological samples, followed by its demethylation and subsequent purification .
化学反応の分析
Types of Reactions
Di-N-desethylamiodarone undergoes various chemical reactions, including:
Oxidation: The compound can be oxidized to form different metabolites.
Reduction: Reduction reactions can modify the functional groups in the molecule.
Substitution: Substitution reactions can occur at various positions on the benzofuran ring
Common Reagents and Conditions
Common reagents used in these reactions include oxidizing agents like hydrogen peroxide and reducing agents like sodium borohydride. The reactions are typically carried out under controlled conditions, such as specific pH levels and temperatures .
Major Products
The major products formed from these reactions include various oxidized and reduced forms of this compound, which can have different pharmacological properties .
科学的研究の応用
Di-N-desethylamiodarone has been extensively studied for its applications in various fields:
作用機序
Di-N-desethylamiodarone exerts its effects by interacting with various molecular targets and pathways:
Potassium Channels: It blocks potassium channels, prolonging the repolarization phase of the cardiac action potential.
Sodium Channels: The compound also blocks sodium channels, affecting the conduction of electrical impulses in the heart.
Adrenergic Receptors: This compound antagonizes alpha- and beta-adrenergic receptors, contributing to its antiarrhythmic effects.
類似化合物との比較
Di-N-desethylamiodarone is compared with other similar compounds, such as:
Mono-N-desethylamiodarone: Another metabolite of amiodarone, which has a single ethyl group removed.
Amiodarone-EtOH: A metabolite formed through the deamination of the diethylaminoethoxy group
Uniqueness
This compound is unique due to its dual demethylation, which imparts distinct pharmacological properties compared to its parent compound and other metabolites. It has been shown to have a greater pro-arrhythmic effect than amiodarone .
特性
IUPAC Name |
[4-(2-aminoethoxy)-3,5-diiodophenyl]-(2-butyl-1-benzofuran-3-yl)methanone | |
---|---|---|
Source | PubChem | |
URL | https://pubchem.ncbi.nlm.nih.gov | |
Description | Data deposited in or computed by PubChem | |
InChI |
InChI=1S/C21H21I2NO3/c1-2-3-7-18-19(14-6-4-5-8-17(14)27-18)20(25)13-11-15(22)21(16(23)12-13)26-10-9-24/h4-6,8,11-12H,2-3,7,9-10,24H2,1H3 | |
Source | PubChem | |
URL | https://pubchem.ncbi.nlm.nih.gov | |
Description | Data deposited in or computed by PubChem | |
InChI Key |
ZOCLVGHDXBVATF-UHFFFAOYSA-N | |
Source | PubChem | |
URL | https://pubchem.ncbi.nlm.nih.gov | |
Description | Data deposited in or computed by PubChem | |
Canonical SMILES |
CCCCC1=C(C2=CC=CC=C2O1)C(=O)C3=CC(=C(C(=C3)I)OCCN)I | |
Source | PubChem | |
URL | https://pubchem.ncbi.nlm.nih.gov | |
Description | Data deposited in or computed by PubChem | |
Molecular Formula |
C21H21I2NO3 | |
Source | PubChem | |
URL | https://pubchem.ncbi.nlm.nih.gov | |
Description | Data deposited in or computed by PubChem | |
DSSTOX Substance ID |
DTXSID90241425 | |
Record name | Di-N-desethylamiodarone | |
Source | EPA DSSTox | |
URL | https://comptox.epa.gov/dashboard/DTXSID90241425 | |
Description | DSSTox provides a high quality public chemistry resource for supporting improved predictive toxicology. | |
Molecular Weight |
589.2 g/mol | |
Source | PubChem | |
URL | https://pubchem.ncbi.nlm.nih.gov | |
Description | Data deposited in or computed by PubChem | |
CAS No. |
94317-95-0 | |
Record name | Di-N-desethylamiodarone | |
Source | ChemIDplus | |
URL | https://pubchem.ncbi.nlm.nih.gov/substance/?source=chemidplus&sourceid=0094317950 | |
Description | ChemIDplus is a free, web search system that provides access to the structure and nomenclature authority files used for the identification of chemical substances cited in National Library of Medicine (NLM) databases, including the TOXNET system. | |
Record name | Di-N-desethylamiodarone | |
Source | EPA DSSTox | |
URL | https://comptox.epa.gov/dashboard/DTXSID90241425 | |
Description | DSSTox provides a high quality public chemistry resource for supporting improved predictive toxicology. | |
Retrosynthesis Analysis
AI-Powered Synthesis Planning: Our tool employs the Template_relevance Pistachio, Template_relevance Bkms_metabolic, Template_relevance Pistachio_ringbreaker, Template_relevance Reaxys, Template_relevance Reaxys_biocatalysis model, leveraging a vast database of chemical reactions to predict feasible synthetic routes.
One-Step Synthesis Focus: Specifically designed for one-step synthesis, it provides concise and direct routes for your target compounds, streamlining the synthesis process.
Accurate Predictions: Utilizing the extensive PISTACHIO, BKMS_METABOLIC, PISTACHIO_RINGBREAKER, REAXYS, REAXYS_BIOCATALYSIS database, our tool offers high-accuracy predictions, reflecting the latest in chemical research and data.
Strategy Settings
Precursor scoring | Relevance Heuristic |
---|---|
Min. plausibility | 0.01 |
Model | Template_relevance |
Template Set | Pistachio/Bkms_metabolic/Pistachio_ringbreaker/Reaxys/Reaxys_biocatalysis |
Top-N result to add to graph | 6 |
Feasible Synthetic Routes
Q1: How does DDEA compare to amiodarone in terms of toxicity to cells?
A: Research suggests that DDEA exhibits lower toxicity towards alveolar macrophages and hepatocytes compared to amiodarone. [, ] This difference in toxicity is attributed to the sequential dealkylation and deamination of the diethylaminoethoxy group present in amiodarone, leading to the formation of DDEA. [] This structural modification appears to be an important detoxification mechanism. []
Q2: What is the significance of DDEA's discovery in understanding amiodarone's activity?
A: Identifying DDEA as a metabolite was crucial in understanding amiodarone's complex pharmacological profile. [] Initially, the unusual pharmacodynamics of amiodarone was thought to be partly influenced by its active metabolites. [] The discovery of DDEA, particularly its presence in the blood of dogs treated with amiodarone and its higher affinity for myocardium compared to the parent drug, provided valuable insights into amiodarone's behavior within the body. []
Q3: Can DDEA be used as a tool to study cellular processes?
A: Interestingly, research has shown that DDEA can be used as a stable isotope label (127I) in combination with imaging techniques like NanoSIMS to visualize and study exocytosis, specifically partial exocytotic content release in cells. [] This approach allowed researchers to track the entry of DDEA into nanovesicles during partial exocytosis, offering valuable insights into this cellular process. []
試験管内研究製品の免責事項と情報
BenchChemで提示されるすべての記事および製品情報は、情報提供を目的としています。BenchChemで購入可能な製品は、生体外研究のために特別に設計されています。生体外研究は、ラテン語の "in glass" に由来し、生物体の外で行われる実験を指します。これらの製品は医薬品または薬として分類されておらず、FDAから任何の医療状態、病気、または疾患の予防、治療、または治癒のために承認されていません。これらの製品を人間または動物に体内に導入する形態は、法律により厳格に禁止されています。これらのガイドラインに従うことは、研究と実験において法的および倫理的な基準の遵守を確実にするために重要です。