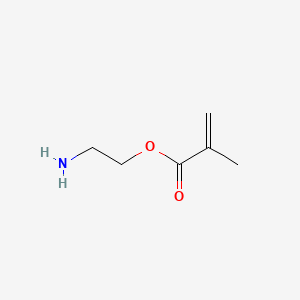
2-Aminoethyl methacrylate
概要
説明
2-Aminoethyl methacrylate (AMA) is an amine-based methacrylic monomer used in the production of polymers and copolymers . It is utilized in a wide range of applications, including coatings, adhesives, and medical devices . It is a highly reactive monomer due to the presence of the methacrylate group, which enables it to undergo both free radical polymerization and other polymerization reactions .
Synthesis Analysis
The synthesis of primary amine-functionalised diblock copolymer nanoparticles via polymerisation-induced self-assembly (PISA) using a RAFT aqueous dispersion polymerisation formulation has been reported . The primary amine steric stabiliser is a macromolecular chain transfer agent (macro-CTA) based on 2-aminoethyl methacrylate AMA .
Molecular Structure Analysis
The molecular formula of 2-Aminoethyl methacrylate is C6H11NO2 . It has a molecular weight of 129.157 Da . It contains total 19 bond(s); 8 non-H bond(s), 2 multiple bond(s), 4 rotatable bond(s), 2 double bond(s), 1 ester(s) (aliphatic), and 1 primary amine(s) (aliphatic) .
Chemical Reactions Analysis
2-Aminoethyl methacrylate is a highly reactive monomer due to the presence of the methacrylate group, which enables it to undergo both free radical polymerization and other polymerization reactions . It can react with various acrylates and acrylamides in aqueous solution at pH 9 .
Physical And Chemical Properties Analysis
2-Aminoethyl methacrylate has a density of 1.0±0.1 g/cm3 . It has a boiling point of 192.1±23.0 °C at 760 mmHg . It has a vapour pressure of 0.5±0.4 mmHg at 25°C . It has a flash point of 63.5±20.1 °C . It is soluble in common organic solvents, very soluble in water, but hydrolyzes slowly .
科学的研究の応用
Regenerative Medicine: Hydrogel Scaffolds
AEMA-modified hyaluronic acid hydrogels have been developed with tunable properties to support the growth and stemness of human dental pulp stem cells (DPSCs). These hydrogels mimic the in vivo 3D environment, which is crucial for maintaining the native spherical morphology and stemness of DPSCs, making them promising for clinical applications in regenerative medicine .
Tissue Engineering: Composite Scaffolds
In tissue engineering, AEMA is used to synthesize polyurethane/urea composite scaffolds. These scaffolds are designed to support the growth and differentiation of cells, which is essential for the development of artificial organs and tissues .
Sensor Applications: Ammonia Gas Sensing
AEMA-based perovskite materials have been investigated for their stability and potential use in ammonia gas sensors. These materials show promise due to their less toxic nature and stability compared to other lead-based perovskite materials .
Dental Applications: Oral Tissue Regeneration
AEMA is utilized in the synthesis of non-cytotoxic hydrogels for dental applications. These hydrogels support the growth of DPSCs and maintain their stemness, which is beneficial for oral tissue regeneration and could lead to advancements in dental treatments .
Biomedical Research: Polymer Synthesis
AEMA is a highly reactive monomer used in the production of polymers and copolymers for various biomedical applications. These include the creation of coatings, adhesives, and medical devices due to its biocompatibility and low toxicity .
Optoelectronics: Perovskite Solar Cells
The stability of AEMA-based perovskite materials is also being explored for use in optoelectronic devices like solar cells. Their enhanced stability and reduced toxicity make them suitable for long-term application in energy-harvesting devices .
作用機序
Target of Action
2-Aminoethyl methacrylate is primarily targeted towards the production of polymers and copolymers . These polymers and copolymers are utilized in a wide range of applications, including coatings, adhesives, and medical devices .
Mode of Action
The compound is a highly reactive monomer due to the presence of the methacrylate group . This enables it to undergo both free radical polymerization and other polymerization reactions . The methacrylate can be polymerized to generate a polymer with pendant amine functionality .
Biochemical Pathways
The compound’s ability to undergo polymerization suggests that it may play a role in the synthesis of polymers for nucleic acid complexation and polyplex formation .
Result of Action
The primary result of 2-Aminoethyl methacrylate’s action is the production of polymers and copolymers with specific properties, such as pendant amine functionality . These polymers and copolymers can be used in various applications, including coatings, adhesives, and medical devices .
Action Environment
The action, efficacy, and stability of 2-Aminoethyl methacrylate can be influenced by environmental factors such as temperature. For instance, the compound is stored at a temperature of 2-8°C . This suggests that temperature control may be important for maintaining the compound’s stability and reactivity.
Safety and Hazards
2-Aminoethyl methacrylate can cause serious eye irritation and skin irritation . It is advised to avoid dust formation, breathing mist, gas or vapours, and contacting with skin and eye . Use of personal protective equipment, wearing chemical impermeable gloves, and ensuring adequate ventilation are recommended .
将来の方向性
The use of 2-Aminoethyl methacrylate in the synthesis of well-defined primary amine-based AB diblock copolymers via atom transfer radical polymerisation (ATRP) has been reported . These copolymers have potential applications in drug delivery, biomedical applications, polypeptide synthesis, and biomineralisation .
特性
IUPAC Name |
2-aminoethyl 2-methylprop-2-enoate | |
---|---|---|
Source | PubChem | |
URL | https://pubchem.ncbi.nlm.nih.gov | |
Description | Data deposited in or computed by PubChem | |
InChI |
InChI=1S/C6H11NO2/c1-5(2)6(8)9-4-3-7/h1,3-4,7H2,2H3 | |
Source | PubChem | |
URL | https://pubchem.ncbi.nlm.nih.gov | |
Description | Data deposited in or computed by PubChem | |
InChI Key |
QLIBJPGWWSHWBF-UHFFFAOYSA-N | |
Source | PubChem | |
URL | https://pubchem.ncbi.nlm.nih.gov | |
Description | Data deposited in or computed by PubChem | |
Canonical SMILES |
CC(=C)C(=O)OCCN | |
Source | PubChem | |
URL | https://pubchem.ncbi.nlm.nih.gov | |
Description | Data deposited in or computed by PubChem | |
Molecular Formula |
C6H11NO2 | |
Source | PubChem | |
URL | https://pubchem.ncbi.nlm.nih.gov | |
Description | Data deposited in or computed by PubChem | |
Related CAS |
26937-52-0 | |
Record name | 2-Propenoic acid, 2-methyl-, 2-aminoethyl ester, homopolymer | |
Source | CAS Common Chemistry | |
URL | https://commonchemistry.cas.org/detail?cas_rn=26937-52-0 | |
Description | CAS Common Chemistry is an open community resource for accessing chemical information. Nearly 500,000 chemical substances from CAS REGISTRY cover areas of community interest, including common and frequently regulated chemicals, and those relevant to high school and undergraduate chemistry classes. This chemical information, curated by our expert scientists, is provided in alignment with our mission as a division of the American Chemical Society. | |
Explanation | The data from CAS Common Chemistry is provided under a CC-BY-NC 4.0 license, unless otherwise stated. | |
DSSTOX Substance ID |
DTXSID60227392 | |
Record name | 2-Aminoethylmethacrylate | |
Source | EPA DSSTox | |
URL | https://comptox.epa.gov/dashboard/DTXSID60227392 | |
Description | DSSTox provides a high quality public chemistry resource for supporting improved predictive toxicology. | |
Molecular Weight |
129.16 g/mol | |
Source | PubChem | |
URL | https://pubchem.ncbi.nlm.nih.gov | |
Description | Data deposited in or computed by PubChem | |
CAS RN |
7659-36-1 | |
Record name | 2-Aminoethylmethacrylate | |
Source | ChemIDplus | |
URL | https://pubchem.ncbi.nlm.nih.gov/substance/?source=chemidplus&sourceid=0007659361 | |
Description | ChemIDplus is a free, web search system that provides access to the structure and nomenclature authority files used for the identification of chemical substances cited in National Library of Medicine (NLM) databases, including the TOXNET system. | |
Record name | 2-Aminoethylmethacrylate | |
Source | EPA DSSTox | |
URL | https://comptox.epa.gov/dashboard/DTXSID60227392 | |
Description | DSSTox provides a high quality public chemistry resource for supporting improved predictive toxicology. | |
Record name | AMINOETHYL METHACRYLATE | |
Source | FDA Global Substance Registration System (GSRS) | |
URL | https://gsrs.ncats.nih.gov/ginas/app/beta/substances/Z4JSA65HUA | |
Description | The FDA Global Substance Registration System (GSRS) enables the efficient and accurate exchange of information on what substances are in regulated products. Instead of relying on names, which vary across regulatory domains, countries, and regions, the GSRS knowledge base makes it possible for substances to be defined by standardized, scientific descriptions. | |
Explanation | Unless otherwise noted, the contents of the FDA website (www.fda.gov), both text and graphics, are not copyrighted. They are in the public domain and may be republished, reprinted and otherwise used freely by anyone without the need to obtain permission from FDA. Credit to the U.S. Food and Drug Administration as the source is appreciated but not required. | |
Synthesis routes and methods
Procedure details
Retrosynthesis Analysis
AI-Powered Synthesis Planning: Our tool employs the Template_relevance Pistachio, Template_relevance Bkms_metabolic, Template_relevance Pistachio_ringbreaker, Template_relevance Reaxys, Template_relevance Reaxys_biocatalysis model, leveraging a vast database of chemical reactions to predict feasible synthetic routes.
One-Step Synthesis Focus: Specifically designed for one-step synthesis, it provides concise and direct routes for your target compounds, streamlining the synthesis process.
Accurate Predictions: Utilizing the extensive PISTACHIO, BKMS_METABOLIC, PISTACHIO_RINGBREAKER, REAXYS, REAXYS_BIOCATALYSIS database, our tool offers high-accuracy predictions, reflecting the latest in chemical research and data.
Strategy Settings
Precursor scoring | Relevance Heuristic |
---|---|
Min. plausibility | 0.01 |
Model | Template_relevance |
Template Set | Pistachio/Bkms_metabolic/Pistachio_ringbreaker/Reaxys/Reaxys_biocatalysis |
Top-N result to add to graph | 6 |
Feasible Synthetic Routes
Q & A
Q1: What is the molecular formula and weight of 2-aminoethyl methacrylate?
A1: The molecular formula of 2-aminoethyl methacrylate is C6H11NO2, and its molecular weight is 129.16 g/mol.
Q2: What spectroscopic techniques are used to characterize 2-aminoethyl methacrylate-based polymers?
A2: Researchers commonly employ proton nuclear magnetic resonance (1H NMR) spectroscopy [, ], carbon nuclear magnetic resonance (13C NMR) spectroscopy [], Fourier-transform infrared (FTIR) spectroscopy [, ], and X-ray photoelectron spectroscopy (XPS) [] to characterize AEMA-based polymers. These techniques provide information about the polymer structure, composition, and functional groups present.
Q3: What polymerization techniques are commonly used for 2-aminoethyl methacrylate?
A3: Several controlled polymerization techniques are employed for AEMA, including atom transfer radical polymerization (ATRP) [, , , , ], reversible addition-fragmentation chain transfer (RAFT) polymerization [, ], and conventional free-radical polymerization []. The choice of technique depends on the desired polymer architecture and properties.
Q4: What are the advantages of using 2-aminoethyl methacrylate in synthesizing materials for biomedical applications?
A4: AEMA's primary amine group allows for easy functionalization and bioconjugation, making it attractive for biomedical applications. It can be used to create biocompatible and biodegradable materials [] suitable for drug delivery [], tissue engineering [, ], and biosensing [].
Q5: How does the incorporation of 2-aminoethyl methacrylate affect the properties of silicone nanoemulsions?
A5: Incorporating AEMA into amphiphilic triblock copolymers used to stabilize silicone nanoemulsions enables charge control []. By co-assembling PAMA-PCL-PAMA (PAMA: poly(2-aminoethyl methacrylate), PCL: poly(ε-caprolactone)) with a zwitterionic triblock copolymer, positive charges can be introduced to the emulsion droplet surface, influencing their stability and interaction with other materials, such as hair [].
Q6: Can 2-aminoethyl methacrylate be used to modify existing materials, and what are the benefits?
A6: Yes, AEMA can modify existing materials, such as porous polypropylene membranes [, ]. Grafting AEMA onto these membranes introduces amine groups, enabling further functionalization for specific applications like metal ion adsorption [] or enzyme immobilization []. This expands the functionality and potential applications of the original material.
Q7: How does the presence of 2-aminoethyl methacrylate in polyplexes influence gene delivery?
A7: AEMA-based polyplexes exhibit excellent potential for gene delivery [, ]. By incorporating AEMA into block copolymers with polyethylene glycol (PEG), researchers achieve enhanced serum tolerance, improved cellular uptake, and efficient DNA release within the target cells []. This highlights the potential of AEMA-based materials for developing effective gene therapies.
Q8: What role does 2-aminoethyl methacrylate play in the development of pH-responsive drug delivery systems?
A8: AEMA is instrumental in creating pH-responsive drug delivery systems [, , ]. Its primary amine group undergoes protonation in acidic environments, causing changes in polymer solubility and charge. This pH sensitivity allows for targeted drug release in acidic tumor microenvironments [, ] or within specific cellular compartments [].
Q9: How does the incorporation of 2-aminoethyl methacrylate affect the thermal properties of polymers?
A9: Incorporating AEMA into polymers can influence their glass transition temperature (Tg), impacting their thermal stability and processing characteristics. For example, methacrylamide-based membranes containing AEMA exhibit higher Tg values (above 100 °C), suppressing unwanted thermal rearrangement and enhancing their performance in CO2 separation applications [].
Q10: How does the stability of poly(2-aminoethyl methacrylate) (PAMA) compare to its monomer, AEMA?
A10: While PAMA is generally more stable than its monomer, it still undergoes slow chemical degradation in alkaline solutions over time []. This degradation involves the elimination of 2-aminoethanol from the polymer backbone []. Careful consideration of pH and storage conditions is crucial for maintaining PAMA stability.
Q11: What strategies can be used to enhance the stability of AEMA-based materials?
A11: Several strategies can improve the stability of AEMA-based materials. One approach involves using protecting groups during synthesis, which can be removed after polymerization []. Another strategy involves incorporating AEMA into block copolymers with more stable polymers like PEG []. Additionally, controlling the pH and storage conditions can minimize degradation [].
Q12: How can the solubility of 2-aminoethyl methacrylate-based polymers be controlled?
A12: The solubility of AEMA-based polymers can be tailored by adjusting the polymer composition, molecular weight, and architecture. For example, incorporating hydrophobic blocks like polystyrene (PS) [] or poly(ε-caprolactone) (PCL) [, ] can create amphiphilic polymers that self-assemble into micelles or vesicles in aqueous solutions.
Q13: What are the advantages of using miniemulsion polymerization for synthesizing 2-aminoethyl methacrylate-based nanoparticles?
A13: Miniemulsion polymerization allows for the controlled synthesis of amino-functionalized nanoparticles with tunable particle size and surface charge []. This method enables the incorporation of AEMA into hydrophobic polymers like styrene or butyl acrylate, creating stable nanoparticles with potential applications in catalysis, sensing, and drug delivery.
Q14: How does the pH value influence the incorporation of AEMA into nanoparticles during miniemulsion polymerization?
A14: Maintaining a pH below 4 during miniemulsion polymerization is crucial for preventing the rearrangement of AEMA into 2-hydroxyethyl methacrylamide []. This acidic environment ensures the stable incorporation of AEMA into the growing polymer chains, yielding amino-functionalized nanoparticles.
Q15: What is the role of 2-aminoethyl methacrylate in developing materials for water remediation?
A15: AEMA plays a crucial role in creating materials for water remediation, particularly in removing heavy metal ions like mercury(II) []. Polymer brushes of poly-AEMA grafted onto magnetic nanoparticles offer a high surface area for functionalization with chelating agents like dithiocarbamate (DTC). These DTC-functionalized MNPs demonstrate impressive efficiency in capturing and removing Hg(II) from contaminated water [].
Q16: How can 2-aminoethyl methacrylate contribute to the development of advanced CO2 capture technologies?
A16: AEMA-containing polymers offer a promising avenue for CO2 capture due to the primary amine groups' ability to react with CO2, forming carbamate species []. This interaction can be harnessed to create "molecular gate" membranes with high selectivity for CO2 over other gases like H2 or He []. This technology holds potential for applications in carbon capture and sequestration, contributing to mitigating climate change.
Q17: What are the potential applications of AEMA-based materials in biosensing?
A17: The versatility of AEMA makes it suitable for various biosensing applications. For instance, immobilizing bioactive peptides onto AEMA-rich copolymer brushes grafted on gold sensors allows for the selective detection of target molecules like immunoglobulin G (IgG) []. These peptide-functionalized surfaces exhibit high sensitivity and selectivity, enabling the development of advanced biosensors for diagnostic and monitoring purposes.
Q18: How can computational chemistry and modeling tools be utilized in AEMA research?
A18: Computational chemistry and modeling techniques are valuable for predicting and understanding the properties and behavior of AEMA-based materials. For example, molecular dynamics simulations can be used to study the self-assembly of AEMA-containing block copolymers in solution, providing insights into their micellar or vesicular structures []. Additionally, quantum chemical calculations can help predict the reactivity of AEMA and its derivatives, guiding the design of novel materials with tailored properties.
試験管内研究製品の免責事項と情報
BenchChemで提示されるすべての記事および製品情報は、情報提供を目的としています。BenchChemで購入可能な製品は、生体外研究のために特別に設計されています。生体外研究は、ラテン語の "in glass" に由来し、生物体の外で行われる実験を指します。これらの製品は医薬品または薬として分類されておらず、FDAから任何の医療状態、病気、または疾患の予防、治療、または治癒のために承認されていません。これらの製品を人間または動物に体内に導入する形態は、法律により厳格に禁止されています。これらのガイドラインに従うことは、研究と実験において法的および倫理的な基準の遵守を確実にするために重要です。