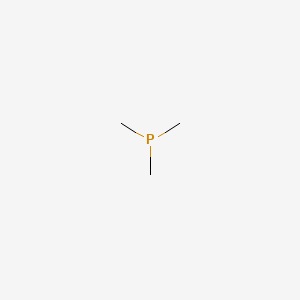
Trimethylphosphine
説明
Trimethylphosphine is an organophosphorus compound with the formula P(CH3)3, commonly abbreviated as PMe3 . This colorless liquid has a strongly unpleasant odor, characteristic of alkylphosphines . The compound is a common ligand in coordination chemistry .
Synthesis Analysis
PMe3 can be prepared by the treatment of triphenyl phosphite with methylmagnesium chloride . The synthesis is conducted in dibutyl ether, from which the more volatile PMe3 can be distilled .Molecular Structure Analysis
Trimethylphosphine is a pyramidal molecule with approximate C3v symmetry . The C–P–C bond angles are approximately 98.6° . The C–P–C bond angles are consistent with the notion that phosphorus predominantly uses the 3p orbitals for forming bonds and that there is little sp hybridization of the phosphorus atom .Chemical Reactions Analysis
With a pKa of 8.65, PMe3 reacts with strong acids to give salts [HPMe3]X . This reaction is reversible . With strong bases, such as alkyl lithium compounds, a methyl group undergoes deprotonation to give PMe2CH2Li .Physical And Chemical Properties Analysis
Trimethylphosphine is a colorless liquid . It has a strongly unpleasant odor, characteristic of alkylphosphines . It is a pyramidal molecule with approximate C3v symmetry . The C–P–C bond angles are approximately 98.6° .科学的研究の応用
Molecular Structure and Bonding :
- Trimethylphosphine (TMP) has been extensively studied for its structural parameters in various states. An electron diffraction study of gaseous TMP revealed detailed structural parameters including bond distances and angles, highlighting its staggered configuration and rotational barriers (Bartell & Brockway, 1960). Similar studies have been conducted on TMP in complex with other elements, providing insights into its molecular structure in different chemical environments (Iijima, Koshimizu, & Shibata, 1981), (Iijima, Hakamata, Nishikawa, & Shibata, 1988).
Catalysis and Chemical Synthesis :
- TMP is used in catalysis, such as in the insertion of acetylenes into carbon-hydrogen bonds catalyzed by rhodium-TMP complexes, highlighting its role in facilitating chemical transformations (Boese & Goldman, 1991). Additionally, it is utilized in the synthesis of various compounds, for instance, in the conversion of ketoximes to ketones (Martín, Martinez, Urpí, & Vilarrasa, 2004).
Nuclear Magnetic Resonance (NMR) Studies :
- The relaxation times of 31P and 13C in TMP have been measured using NMR, revealing insights into the molecular dynamics and motion of TMP. These studies contribute to our understanding of the anisotropic motion of TMP molecules at various temperatures (Robert, Taieb, & Tabony, 1980).
Surface Science and Material Modification :
- TMP has been used to study the adsorption characteristics on surfaces like Si(111)-(7 × 7), where it shows selective adsorption patterns. This is significant for understanding surface chemistry and material modifications (Shimomura, Sanada, Fukuda, & M∅ller, 1995). It also plays a role in modifying zeolite materials, as seen in its interaction with HZSM-5 zeolites (Rumplmayr & Lercher, 1990).
Biochemical Applications :
- In biochemistry, TMP has been used as a probe for studying hemoglobin and myoglobin, providing valuable information about the perturbations in the beta heme pocket induced by a thiol reagent (Bondon, Sodano, Simonneaux, & Craescu, 1987).
作用機序
Target of Action
Trimethylphosphine, also known as PMe3, is an organophosphorus compound . It is an electron-rich phosphine ligand , which means it primarily targets metal ions in its reactions. It forms complexes with most metals , serving as a common ligand in coordination chemistry .
Mode of Action
Trimethylphosphine interacts with its targets (metal ions) by donating its lone pair of electrons to form a coordinate bond . This interaction results in the formation of metal-phosphine complexes . The compound’s Tolman cone angle, an indication of the steric protection it provides to the metal it is bound to, is 118° .
Biochemical Pathways
It is known to be used in various chemical reactions, including the mitsunobu reaction . It can also be used in the transformation of azides into carbamates, aziridines to azidoalcohols, and ketoximes to ketones with 2,2′-dipyridyl diselenide .
Result of Action
The primary result of trimethylphosphine’s action is the formation of metal-phosphine complexes . These complexes are often used as catalysts in various chemical reactions .
Action Environment
The action, efficacy, and stability of trimethylphosphine can be influenced by various environmental factors. For instance, its reactivity can be affected by the presence of strong acids or bases . It is also easily oxidized to the phosphine oxide with oxygen .
Safety and Hazards
特性
IUPAC Name |
trimethylphosphane | |
---|---|---|
Source | PubChem | |
URL | https://pubchem.ncbi.nlm.nih.gov | |
Description | Data deposited in or computed by PubChem | |
InChI |
InChI=1S/C3H9P/c1-4(2)3/h1-3H3 | |
Source | PubChem | |
URL | https://pubchem.ncbi.nlm.nih.gov | |
Description | Data deposited in or computed by PubChem | |
InChI Key |
YWWDBCBWQNCYNR-UHFFFAOYSA-N | |
Source | PubChem | |
URL | https://pubchem.ncbi.nlm.nih.gov | |
Description | Data deposited in or computed by PubChem | |
Canonical SMILES |
CP(C)C | |
Source | PubChem | |
URL | https://pubchem.ncbi.nlm.nih.gov | |
Description | Data deposited in or computed by PubChem | |
Molecular Formula |
C3H9P | |
Source | PubChem | |
URL | https://pubchem.ncbi.nlm.nih.gov | |
Description | Data deposited in or computed by PubChem | |
DSSTOX Substance ID |
DTXSID00208120 | |
Record name | Trimethyl phosphine | |
Source | EPA DSSTox | |
URL | https://comptox.epa.gov/dashboard/DTXSID00208120 | |
Description | DSSTox provides a high quality public chemistry resource for supporting improved predictive toxicology. | |
Molecular Weight |
76.08 g/mol | |
Source | PubChem | |
URL | https://pubchem.ncbi.nlm.nih.gov | |
Description | Data deposited in or computed by PubChem | |
Physical Description |
Colorless liquid with a pungent odor; [Alfa Aesar MSDS] | |
Record name | Trimethylphosphine | |
Source | Haz-Map, Information on Hazardous Chemicals and Occupational Diseases | |
URL | https://haz-map.com/Agents/18372 | |
Description | Haz-Map® is an occupational health database designed for health and safety professionals and for consumers seeking information about the adverse effects of workplace exposures to chemical and biological agents. | |
Explanation | Copyright (c) 2022 Haz-Map(R). All rights reserved. Unless otherwise indicated, all materials from Haz-Map are copyrighted by Haz-Map(R). No part of these materials, either text or image may be used for any purpose other than for personal use. Therefore, reproduction, modification, storage in a retrieval system or retransmission, in any form or by any means, electronic, mechanical or otherwise, for reasons other than personal use, is strictly prohibited without prior written permission. | |
Product Name |
Trimethylphosphine | |
CAS RN |
594-09-2 | |
Record name | Trimethylphosphine | |
Source | CAS Common Chemistry | |
URL | https://commonchemistry.cas.org/detail?cas_rn=594-09-2 | |
Description | CAS Common Chemistry is an open community resource for accessing chemical information. Nearly 500,000 chemical substances from CAS REGISTRY cover areas of community interest, including common and frequently regulated chemicals, and those relevant to high school and undergraduate chemistry classes. This chemical information, curated by our expert scientists, is provided in alignment with our mission as a division of the American Chemical Society. | |
Explanation | The data from CAS Common Chemistry is provided under a CC-BY-NC 4.0 license, unless otherwise stated. | |
Record name | Trimethyl phosphine | |
Source | ChemIDplus | |
URL | https://pubchem.ncbi.nlm.nih.gov/substance/?source=chemidplus&sourceid=0000594092 | |
Description | ChemIDplus is a free, web search system that provides access to the structure and nomenclature authority files used for the identification of chemical substances cited in National Library of Medicine (NLM) databases, including the TOXNET system. | |
Record name | Trimethyl phosphine | |
Source | EPA DSSTox | |
URL | https://comptox.epa.gov/dashboard/DTXSID00208120 | |
Description | DSSTox provides a high quality public chemistry resource for supporting improved predictive toxicology. | |
Record name | Trimethylphosphine | |
Source | European Chemicals Agency (ECHA) | |
URL | https://echa.europa.eu/substance-information/-/substanceinfo/100.008.932 | |
Description | The European Chemicals Agency (ECHA) is an agency of the European Union which is the driving force among regulatory authorities in implementing the EU's groundbreaking chemicals legislation for the benefit of human health and the environment as well as for innovation and competitiveness. | |
Explanation | Use of the information, documents and data from the ECHA website is subject to the terms and conditions of this Legal Notice, and subject to other binding limitations provided for under applicable law, the information, documents and data made available on the ECHA website may be reproduced, distributed and/or used, totally or in part, for non-commercial purposes provided that ECHA is acknowledged as the source: "Source: European Chemicals Agency, http://echa.europa.eu/". Such acknowledgement must be included in each copy of the material. ECHA permits and encourages organisations and individuals to create links to the ECHA website under the following cumulative conditions: Links can only be made to webpages that provide a link to the Legal Notice page. | |
Record name | TRIMETHYL PHOSPHINE | |
Source | FDA Global Substance Registration System (GSRS) | |
URL | https://gsrs.ncats.nih.gov/ginas/app/beta/substances/5FL6SQK9H3 | |
Description | The FDA Global Substance Registration System (GSRS) enables the efficient and accurate exchange of information on what substances are in regulated products. Instead of relying on names, which vary across regulatory domains, countries, and regions, the GSRS knowledge base makes it possible for substances to be defined by standardized, scientific descriptions. | |
Explanation | Unless otherwise noted, the contents of the FDA website (www.fda.gov), both text and graphics, are not copyrighted. They are in the public domain and may be republished, reprinted and otherwise used freely by anyone without the need to obtain permission from FDA. Credit to the U.S. Food and Drug Administration as the source is appreciated but not required. | |
Retrosynthesis Analysis
AI-Powered Synthesis Planning: Our tool employs the Template_relevance Pistachio, Template_relevance Bkms_metabolic, Template_relevance Pistachio_ringbreaker, Template_relevance Reaxys, Template_relevance Reaxys_biocatalysis model, leveraging a vast database of chemical reactions to predict feasible synthetic routes.
One-Step Synthesis Focus: Specifically designed for one-step synthesis, it provides concise and direct routes for your target compounds, streamlining the synthesis process.
Accurate Predictions: Utilizing the extensive PISTACHIO, BKMS_METABOLIC, PISTACHIO_RINGBREAKER, REAXYS, REAXYS_BIOCATALYSIS database, our tool offers high-accuracy predictions, reflecting the latest in chemical research and data.
Strategy Settings
Precursor scoring | Relevance Heuristic |
---|---|
Min. plausibility | 0.01 |
Model | Template_relevance |
Template Set | Pistachio/Bkms_metabolic/Pistachio_ringbreaker/Reaxys/Reaxys_biocatalysis |
Top-N result to add to graph | 6 |
Feasible Synthetic Routes
試験管内研究製品の免責事項と情報
BenchChemで提示されるすべての記事および製品情報は、情報提供を目的としています。BenchChemで購入可能な製品は、生体外研究のために特別に設計されています。生体外研究は、ラテン語の "in glass" に由来し、生物体の外で行われる実験を指します。これらの製品は医薬品または薬として分類されておらず、FDAから任何の医療状態、病気、または疾患の予防、治療、または治癒のために承認されていません。これらの製品を人間または動物に体内に導入する形態は、法律により厳格に禁止されています。これらのガイドラインに従うことは、研究と実験において法的および倫理的な基準の遵守を確実にするために重要です。