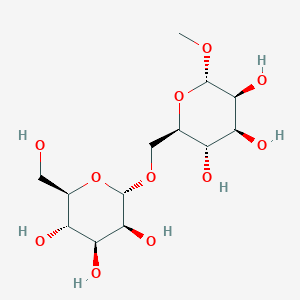
Methyl 6-O-(a-D-mannopyranosyl)-a-D-mannopyranoside
説明
Methyl 6-O-(α-D-mannopyranosyl)-α-D-mannopyranoside is a disaccharide derivative in which an α-D-mannopyranosyl unit is linked via a glycosidic bond to the 6-hydroxyl group of a methyl α-D-mannopyranoside core. This compound is pivotal in glycobiology for studying carbohydrate-protein interactions, particularly in lectin binding and immune response modulation . Its synthesis typically involves regioselective acylation or glycosylation of methyl α-D-mannopyranoside precursors. For example, describes the synthesis of methyl 6-O-p-toluoyl-α-D-mannopyranoside (a related acylated derivative) using p-toluoyl chloride under controlled conditions, achieving high yields . The compound’s structure is confirmed via FTIR, NMR, and mass spectrometry .
特性
IUPAC Name |
(2R,3S,4S,5S,6S)-2-(hydroxymethyl)-6-[[(2R,3S,4S,5S,6S)-3,4,5-trihydroxy-6-methoxyoxan-2-yl]methoxy]oxane-3,4,5-triol | |
---|---|---|
Source | PubChem | |
URL | https://pubchem.ncbi.nlm.nih.gov | |
Description | Data deposited in or computed by PubChem | |
InChI |
InChI=1S/C13H24O11/c1-21-12-10(19)9(18)7(16)5(24-12)3-22-13-11(20)8(17)6(15)4(2-14)23-13/h4-20H,2-3H2,1H3/t4-,5-,6-,7-,8+,9+,10+,11+,12+,13+/m1/s1 | |
Source | PubChem | |
URL | https://pubchem.ncbi.nlm.nih.gov | |
Description | Data deposited in or computed by PubChem | |
InChI Key |
ZQPVHVKWCGZNDW-OGVZYCONSA-N | |
Source | PubChem | |
URL | https://pubchem.ncbi.nlm.nih.gov | |
Description | Data deposited in or computed by PubChem | |
Canonical SMILES |
COC1C(C(C(C(O1)COC2C(C(C(C(O2)CO)O)O)O)O)O)O | |
Source | PubChem | |
URL | https://pubchem.ncbi.nlm.nih.gov | |
Description | Data deposited in or computed by PubChem | |
Isomeric SMILES |
CO[C@@H]1[C@H]([C@H]([C@@H]([C@H](O1)CO[C@@H]2[C@H]([C@H]([C@@H]([C@H](O2)CO)O)O)O)O)O)O | |
Source | PubChem | |
URL | https://pubchem.ncbi.nlm.nih.gov | |
Description | Data deposited in or computed by PubChem | |
Molecular Formula |
C13H24O11 | |
Source | PubChem | |
URL | https://pubchem.ncbi.nlm.nih.gov | |
Description | Data deposited in or computed by PubChem | |
DSSTOX Substance ID |
DTXSID001000164 | |
Record name | Methyl 6-O-hexopyranosylhexopyranoside | |
Source | EPA DSSTox | |
URL | https://comptox.epa.gov/dashboard/DTXSID001000164 | |
Description | DSSTox provides a high quality public chemistry resource for supporting improved predictive toxicology. | |
Molecular Weight |
356.32 g/mol | |
Source | PubChem | |
URL | https://pubchem.ncbi.nlm.nih.gov | |
Description | Data deposited in or computed by PubChem | |
CAS No. |
78962-39-7 | |
Record name | Methyl 6-O-mannopyranosylmannopyranoside | |
Source | ChemIDplus | |
URL | https://pubchem.ncbi.nlm.nih.gov/substance/?source=chemidplus&sourceid=0078962397 | |
Description | ChemIDplus is a free, web search system that provides access to the structure and nomenclature authority files used for the identification of chemical substances cited in National Library of Medicine (NLM) databases, including the TOXNET system. | |
Record name | Methyl 6-O-hexopyranosylhexopyranoside | |
Source | EPA DSSTox | |
URL | https://comptox.epa.gov/dashboard/DTXSID001000164 | |
Description | DSSTox provides a high quality public chemistry resource for supporting improved predictive toxicology. | |
準備方法
Two-Step Deprotection and Hydrogenolysis
Reaction Sequence and Conditions
The most direct synthesis involves a two-step protocol starting from a fully protected precursor. Methyl 2-O-acetyl-3,4,6-tri-O-benzyl-α-D-mannopyranosyl-(1→6)-2,3,4-tri-O-benzyl-α-D-mannopyranoside undergoes sequential deprotection:
-
Base-Catalyzed Acetyl Removal : Treatment with sodium methoxide (NaOMe) in methanol at 20°C for 24 hours under nitrogen cleaves the 2-O-acetyl group.
-
Hydrogenolytic Debenzylation : Palladium on carbon (Pd/C, 10 wt%) and hydrogen gas in methanol/acetic acid (20°C, 72 hours) remove benzyl protecting groups.
Yield and Purification
Flash column chromatography (DCM:MeOH, 3:1) yields 77% of the target compound. Key spectral data confirm success:
-
¹H-NMR (500 MHz, D₂O) : δ 4.90 (d, J = 1.6 Hz, H-1’), 4.74 (d, J = 1.6 Hz, H-1), 3.40 ppm (s, OMe).
-
¹³C-NMR (125 MHz, D₂O) : 101.6 (C-1’), 100.1 (C-1), 55.4 ppm (OMe).
Advantages and Limitations
-
Advantages : High regioselectivity, straightforward purification.
-
Limitations : Prolonged hydrogenolysis (72 hours) and sensitivity to residual acetic acid.
Helferich Glycosylation with Acetylated Mannosyl Bromide
Glycosylation Strategy
This method employs Helferich glycosylation using 2,3,4,6-tetra-O-acetyl-α-D-mannopyranosyl bromide:
-
First Glycosylation : Methyl 2-O-allyl-4,6-O-benzylidene-α-D-mannopyranoside reacts with the bromide under catalysis (e.g., Hg(CN)₂), yielding a 3-O-glycosylated intermediate (90% yield).
-
Benzylidene Removal : Acidic hydrolysis (e.g., HCl in dioxane) exposes the 6-OH group.
-
Second Glycosylation : Repeat glycosylation at the 6-position.
-
Final Deprotection : Zemplén deacetylation (NaOMe/MeOH) and deallylation (PdCl₂/NaOAc) yield the target compound.
Silver Triflate-Mediated Glycosylation
Activation of Glycosyl Donors
Silver trifluoromethanesulfonate (AgOTf) and tetramethylurea (TMU) promote glycosylation between methyl 2,3,4-tri-O-benzyl-α-D-mannopyranoside and peracetylated mannosyl bromides:
-
Conditions : Anhydrous DCM, 0°C to room temperature, 4–6 hours.
-
Outcome : High α-selectivity (>90%) due to neighboring group participation from acetyl groups.
Deprotection Protocol
-
Benzyl Removal : Hydrogenolysis (Pd/C, H₂, 48 hours).
-
Acetyl Removal : Zemplén conditions (NaOMe/MeOH).
Thioglycoside Activation for Convergent Synthesis
Comparative Analysis of Methods
Structural Validation Techniques
Nuclear Magnetic Resonance (NMR)
Challenges and Optimization Strategies
Regioselectivity in Glycosylation
Mannose’s 2-, 3-, and 6-hydroxyls present competing reaction sites. Strategies to enhance 6-O-selectivity include:
化学反応の分析
Types of Reactions
Methyl 6-O-(a-D-mannopyranosyl)-a-D-mannopyranoside can undergo various chemical reactions, including:
Oxidation: This reaction can convert the hydroxyl groups into carbonyl groups.
Reduction: The compound can be reduced to form different sugar alcohols.
Substitution: The hydroxyl groups can be substituted with other functional groups using appropriate reagents.
Common Reagents and Conditions
Oxidation: Reagents like periodic acid or bromine water.
Reduction: Sodium borohydride or lithium aluminum hydride.
Substitution: Acyl chlorides or alkyl halides under basic conditions.
Major Products
The major products formed from these reactions include oxidized derivatives, reduced sugar alcohols, and substituted glycosides .
科学的研究の応用
Chemical Applications
Building Block in Synthesis:
Methyl 6-O-(α-D-mannopyranosyl)-α-D-mannopyranoside serves as a crucial building block in the synthesis of complex carbohydrates and glycosides. Its structural features make it suitable for creating various glycosidic linkages, which are essential in the development of oligosaccharides and polysaccharides used in research and industrial applications.
Table 1: Synthesis Applications of Methyl 6-O-(α-D-mannopyranosyl)-α-D-mannopyranoside
Biological Applications
Carbohydrate-Protein Interactions:
The compound is extensively studied for its role in carbohydrate-protein interactions. It acts as a substrate for glycosidase enzymes, facilitating research into enzyme specificity and kinetics. Its ability to mimic natural substrates allows researchers to explore mechanisms of enzyme action and inhibition.
Antimicrobial Properties:
Recent studies have highlighted the potential of methyl 6-O-(α-D-mannopyranosyl)-α-D-mannopyranoside derivatives in antimicrobial applications. For instance, derivatives have shown promising antifungal properties against several strains while maintaining low cytotoxicity levels. This positions them as candidates for developing new antimicrobial agents.
Table 2: Biological Studies Involving Methyl 6-O-(α-D-mannopyranosyl)-α-D-mannopyranoside
Medical Applications
Drug Delivery Systems:
Methyl 6-O-(α-D-mannopyranosyl)-α-D-mannopyranoside is being investigated for its potential role in drug delivery systems, particularly for targeting specific cell types such as macrophages. The ability to modify nanoparticles with mannose residues enhances their uptake by cells expressing mannose receptors, making them effective carriers for therapeutic agents.
Therapeutic Potential:
The compound's interactions with various biomolecules suggest potential therapeutic applications. Research into its effects on cellular processes indicates that it may influence gene expression and cellular metabolism, which could be leveraged for therapeutic interventions.
Table 3: Medical Research Applications
Industrial Applications
Biodegradable Materials:
In industrial settings, methyl 6-O-(α-D-mannopyranosyl)-α-D-mannopyranoside is utilized in the production of biodegradable materials. Its properties make it suitable as a stabilizer in various formulations, contributing to the development of environmentally friendly products.
Stabilizer in Formulations:
The compound's ability to interact with other components allows it to function effectively as a stabilizer, enhancing the shelf-life and performance of products across different industries.
Case Studies
-
Targeting Macrophages in Tuberculosis Therapy:
A study explored the use of mannosylated solid lipid nanoparticles engineered with methyl α-D-mannopyranoside to enhance drug delivery specifically to infected macrophages in tuberculosis treatment. The results indicated improved targeting efficiency and therapeutic outcomes (). -
Antimicrobial Derivatives Development:
Research focused on synthesizing cinnamoyl-substituted derivatives of methyl α-D-mannopyranoside demonstrated significant antimicrobial activity against various pathogens while revealing favorable molecular docking profiles against the H5N1 influenza A virus ().
作用機序
The mechanism of action of Methyl 6-O-(a-D-mannopyranosyl)-a-D-mannopyranoside involves its interaction with specific molecular targets, such as lectins and glycosidases. The compound can inhibit or enhance the activity of these enzymes, affecting various biochemical pathways. Its glycosidic bond plays a crucial role in its binding affinity and specificity .
類似化合物との比較
Methyl 6-O-(α-D-mannopyranosyl)-α-D-mannopyranoside belongs to a broader class of mannopyranoside derivatives. Below is a detailed comparison with structurally and functionally related compounds:
Structural Analogues with Varied Substituents
Key Findings :
- Acylated Derivatives: Compounds like methyl 2,3,4-tri-O-octanoyl-α-D-mannopyranoside exhibit increased lipophilicity, enhancing membrane permeability and antimicrobial efficacy compared to the parent compound .
- Aryl Derivatives : The 4-nitrophenyl analogue’s chromogenic properties make it superior for enzymatic assays, whereas the methyl derivative is more suited for lectin-binding studies due to its natural aglycone .
Isomeric and Linkage Variants
Key Findings :
- Linkage Position : The 6-O-linked derivative shows stronger binding to concanavalin A than 1→2 or 1→3 analogues, as the 6-position allows optimal interaction with lectin binding pockets .
- Branching Effects: Trisaccharide derivatives (e.g., 3,6-di-O-mannosyl) mimic high-mannose N-glycans, making them valuable for immunological studies .
Functional Derivatives in Biomedical Research
Key Findings :
- Sulfonamide Derivatives : The introduction of sulfanilyl groups (e.g., in compound 7, ) enhances antibacterial activity by targeting bacterial enzymes .
生物活性
Chemical Structure and Properties
M6-O-MM is a complex carbohydrate derivative composed of two mannose units linked through a glycosidic bond. Its molecular formula is . The compound's unique structural properties allow it to interact with various biological systems, particularly in modulating carbohydrate metabolism and glycosylation processes.
Target Interactions
M6-O-MM exhibits significant affinity for specific receptors involved in cellular signaling pathways. Notably, it competes with mannose for binding to the FimH lectin found on uropathogenic Escherichia coli (UPEC), which plays a crucial role in bacterial adhesion to urothelial cells. This interaction is vital for the bacteria’s ability to cause urinary tract infections (UTIs) .
Mode of Action
- Inhibition of Bacterial Adhesion : M6-O-MM has been shown to inhibit the binding of mannose by E. coli, thereby disrupting its normal function and potentially reducing bacterial growth or survival .
- Cell Signaling Modulation : By interacting with various enzymes and proteins, M6-O-MM influences cell signaling pathways, gene expression, and cellular metabolism .
Antimicrobial Properties
Research indicates that M6-O-MM has promising antimicrobial activities:
- Inhibition of UPEC : Studies have demonstrated that M6-O-MM can effectively block UPEC adhesion to bladder cells, thereby reducing bacterial load in murine models of cystitis .
- Broad-Spectrum Antimicrobial Activity : In vitro assays have shown that derivatives of mannopyranosides exhibit significant antibacterial and antifungal properties against various strains, including Staphylococcus aureus and Bacillus cereus .
Cytotoxicity and Safety Profiles
The cytotoxic effects of M6-O-MM have been evaluated in several studies. For example, derivatives synthesized from M6-O-MM displayed low toxicity levels in HepG2 human liver cells, indicating their potential as safe therapeutic agents . The toxicity profiles are crucial for considering their application in drug development.
Table 1: Summary of Biological Activities
Case Study: Inhibition of UPEC Adhesion
A systematic study evaluated the efficacy of M6-O-MM against UPEC in a murine model. The results indicated that administration of M6-O-MM significantly reduced bacterial colonization in the bladder compared to control groups. This supports its potential use as a therapeutic agent against UTIs.
Pharmacokinetics and Metabolism
M6-O-MM exhibits favorable pharmacokinetic properties, including good solubility in water, which enhances its bioavailability. Its metabolic pathways involve interactions with various enzymes that modulate its activity within biological systems .
Q & A
Basic Research Questions
Q. What are the established synthetic routes for Methyl 6-O-(α-D-mannopyranosyl)-α-D-mannopyranoside, and how do reaction conditions influence glycosylation efficiency?
- Answer : The compound is typically synthesized via glycosylation reactions using protected mannopyranosyl donors. For example, methyl α-D-mannopyranoside derivatives are activated with agents like N-iodosuccinimide (NIS) and AgOTf in dichloromethane (DCM) at low temperatures (-30°C) to promote stereoselective α-linkage formation . Protecting groups such as benzyl or benzoyl are critical to prevent undesired side reactions. Reaction efficiency depends on donor-acceptor ratios, solvent polarity, and activation methods. For instance, molecular sieves are often used to scavenge moisture and improve yields .
Q. How is the purity and structural identity of this compound verified in synthetic workflows?
- Answer : Post-synthesis purification involves column chromatography (silica gel or reverse-phase C18 cartridges) and crystallization. Structural confirmation relies on:
- NMR spectroscopy : Key signals include anomeric protons (δ ~4.8–5.3 ppm, ~1.5–3.3 Hz for α-linkages) and methyl group resonances (δ ~3.4 ppm) .
- Mass spectrometry : ESI-MS or HRMS validates molecular weight (e.g., [M + Na]+ calc. 379.1211 for disaccharides) .
- Polarimetry : Specific rotation ([α]D) confirms stereochemical integrity (e.g., +26.04° for α-linked mannosides) .
Q. What challenges arise during the synthesis of branched oligosaccharides containing this disaccharide unit?
- Answer : Key challenges include:
- Regioselectivity : Competing reactions at C2, C3, or C6 hydroxyl groups require careful protecting group strategies (e.g., benzyl vs. benzoyl groups) .
- Steric hindrance : Bulky substituents (e.g., trityl groups) at C6 can slow glycosylation kinetics, necessitating extended reaction times .
- Deprotection : Hydrogenolysis (Pd(OH)₂/C) or basic hydrolysis (NaOMe) must preserve acid-sensitive glycosidic bonds .
Advanced Research Questions
Q. How do nuclear Overhauser effect (NOE) correlations and -labeling aid in resolving the conformational dynamics of this compound?
- Answer : -isotopic labeling (e.g., at C6) combined with NOESY experiments reveals spatial proximity between anomeric protons and adjacent residues, confirming linkage stereochemistry and ring puckering . For example, coupling constants (~170–172 Hz for C1) differentiate α- and β-anomers . Computational modeling (DFT or MD simulations) further predicts low-energy conformers, validated by NMR-derived interproton distances .
Q. What mechanistic insights explain contradictory glycosylation outcomes in analogous mannoside derivatives?
- Answer : Discrepancies in glycosylation efficiency (e.g., α vs. β selectivity) arise from:
- Donor leaving groups : Thioglycosides (e.g., ethyl 1-thio-mannosides) offer better control than halides due to slower activation kinetics .
- Solvent effects : Polar aprotic solvents (DCM) favor SN2-like mechanisms for β-linkages, while less polar media stabilize oxocarbenium intermediates for α-selectivity .
- Additives : Silver triflate (AgOTf) stabilizes glycosyl triflates, promoting α-stereochemistry via ion-pair intermediates .
Q. How does Methyl 6-O-(α-D-mannopyranosyl)-α-D-mannopyranoside interact with lectins like concanavalin A (ConA), and what are the implications for glycobiology?
- Answer : The compound binds ConA via its terminal α-1,6-mannose residues, as shown by X-ray crystallography and isothermal titration calorimetry (ITC). The binding site involves Tyr-12, Asp-16, and Asp-208 residues, with a dissociation constant () in the micromolar range . This interaction mimics natural glycoprotein recognition, aiding studies on immune response modulation and pathogen-host adhesion .
Q. What advanced mass spectrometry techniques are employed to analyze heterogeneous glycosylation patterns in derivatives of this compound?
- Answer : High-resolution MALDI-TOF or LC-ESI-MS/MS with collision-induced dissociation (CID) fragments glycosidic bonds, enabling sequencing of branched structures. For example, -labeled analogs reduce spectral complexity by distinguishing natural isotopes from synthetic labels . Ion mobility spectrometry (IMS) further separates isomeric glycans based on drift time differences .
Q. Methodological Considerations
Q. How are contradictory NMR assignments for closely related mannosides resolved?
- Answer : Ambiguities in proton assignments (e.g., H2 vs. H3) are addressed via:
- 2D-COSY/TOCSY : Correlates scalar-coupled spins within the sugar ring.
- HSQC/HMBC : Links - correlations across glycosidic bonds.
- Comparative analysis : Benchmarking against known standards (e.g., phyproof® reference substances) .
Q. What strategies optimize the synthesis of isotopically labeled analogs for metabolic tracing or structural studies?
Retrosynthesis Analysis
AI-Powered Synthesis Planning: Our tool employs the Template_relevance Pistachio, Template_relevance Bkms_metabolic, Template_relevance Pistachio_ringbreaker, Template_relevance Reaxys, Template_relevance Reaxys_biocatalysis model, leveraging a vast database of chemical reactions to predict feasible synthetic routes.
One-Step Synthesis Focus: Specifically designed for one-step synthesis, it provides concise and direct routes for your target compounds, streamlining the synthesis process.
Accurate Predictions: Utilizing the extensive PISTACHIO, BKMS_METABOLIC, PISTACHIO_RINGBREAKER, REAXYS, REAXYS_BIOCATALYSIS database, our tool offers high-accuracy predictions, reflecting the latest in chemical research and data.
Strategy Settings
Precursor scoring | Relevance Heuristic |
---|---|
Min. plausibility | 0.01 |
Model | Template_relevance |
Template Set | Pistachio/Bkms_metabolic/Pistachio_ringbreaker/Reaxys/Reaxys_biocatalysis |
Top-N result to add to graph | 6 |
Feasible Synthetic Routes
Featured Recommendations
Most viewed | ||
---|---|---|
Most popular with customers |
試験管内研究製品の免責事項と情報
BenchChemで提示されるすべての記事および製品情報は、情報提供を目的としています。BenchChemで購入可能な製品は、生体外研究のために特別に設計されています。生体外研究は、ラテン語の "in glass" に由来し、生物体の外で行われる実験を指します。これらの製品は医薬品または薬として分類されておらず、FDAから任何の医療状態、病気、または疾患の予防、治療、または治癒のために承認されていません。これらの製品を人間または動物に体内に導入する形態は、法律により厳格に禁止されています。これらのガイドラインに従うことは、研究と実験において法的および倫理的な基準の遵守を確実にするために重要です。