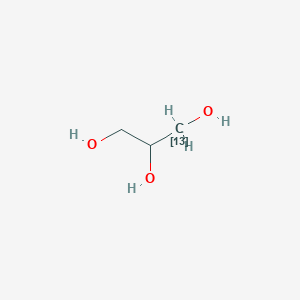
Glycerol-13C
説明
Glycerol-13C is a stable isotope-labeled derivative of glycerol, where one or more carbon atoms are replaced with carbon-13 (13C). This compound is widely utilized in metabolic studies, nuclear magnetic resonance (NMR) spectroscopy, and isotopic tracing to investigate biochemical pathways. For example, glycerol-1,3-13C2 (CAS: 102088-01-7) features 13C labeling at the 1st and 3rd carbon positions, enabling precise tracking of glycerol metabolism in vivo . Its applications span pharmacokinetics, lipid metabolism research, and dynamic nuclear polarization (DNP) experiments, where its longitudinal relaxation (T1) properties are critical for enhancing NMR sensitivity .
準備方法
Chemical Synthesis and NMR-Based Quantification
The preparation of glycerol-13C often begins with chemical synthesis using 13C-enriched precursors, followed by rigorous characterization via 1H and 13C NMR spectroscopy. A validated NMR method for glycerol quantification in drug formulations demonstrates the utility of isotopic labeling for analytical precision . In this approach, this compound is synthesized by substituting natural-abundance carbon sources with 13C-labeled substrates during esterification or reduction reactions. For example, reducing 13C-labeled glucose or glyceraldehyde with sodium borohydride yields this compound with specific isotopic enrichment at designated carbon positions.
NMR Spectral Assignments and Structural Confirmation
1H and 13C NMR spectra of this compound reveal distinct chemical shifts that differ from unlabeled glycerol due to isotopic effects. As shown in Table 1, the proton resonances of glycerol appear at δ 3.52–3.77 ppm, while its carbon signals occur at δ 62.5 (methylene) and δ 73.1 (methine) . The incorporation of 13C alters spin-spin coupling patterns, enabling unambiguous identification. For instance, the 13C-enriched methine group at δ 73.1 exhibits split peaks in 1H NMR due to 13C-1H coupling, a feature absent in natural-abundance glycerol .
Table 1: NMR Chemical Shifts for Glycerol and Analogous Compounds
Compound | 1H Shifts (ppm) | 13C Shifts (ppm) |
---|---|---|
This compound | 3.52–3.77 (m, dd) | 62.5, 73.1 |
1,2-Propanediol | 3.44–3.86 (m) | 17.9–67.9 |
Maleic Acid | 6.40 (s) | 132.6, 170.8 |
Validation of Synthetic Purity
Quantitative NMR (qNMR) methods ensure the purity and concentration of synthesized this compound. Using maleic acid as an internal standard, the linear regression equation y = 20.912x − 0.435 (r² = 1.0000) correlates the integration ratio of this compound to maleic acid in 1H NMR . The limit of detection (LOD) and quantification (LOQ) for this method are 0.015 mM and 0.045 mM, respectively, surpassing the sensitivity of traditional titration methods . Notably, 13C NMR achieves comparable accuracy but requires longer acquisition times due to lower natural abundance of 13C nuclei .
Metabolic Labeling in Biological Systems
In vivo production of this compound through metabolic labeling offers a biologically relevant alternative to chemical synthesis. The green alga Chlamydomonas reinhardtii has been employed to generate 13C-labeled glycerol as part of lipid remodeling under nitrogen deprivation .
Isotopic Precursor Incorporation
Feeding C. reinhardtii with [13C2]acetate results in the incorporation of 13C into glycerol backbones of membrane lipids such as monogalactosyldiacylglycerol (MGDG) and digalactosyldiacylglycerol (DGDG) . During nitrogen deprivation, these lipids undergo hydrolysis, releasing 13C-labeled glycerol and fatty acids into the triacylglycerol (TAG) pool. Approximately 33% of glycerol moieties in TAG originate from preexisting membrane lipids, as determined by pulse-chase 13C-labeling experiments .
Turnover Dynamics and Lipid Recycling
Gas chromatography–mass spectrometry (GC–MS) and ultra-high performance liquid chromatography–tandem MS (UPLC-MS/MS) analyses reveal synchronous turnover of glycerol backbones and headgroups in galactolipids . For example, the 18:3α/16:4 MGDG species contributes glyceryl moieties to TAG via headgroup removal, a process mediated by phospholipase A1 (PLA1) and phosphatidylcholine:diacylglycerol cholinephosphotransferase (PDAT) . This metabolic route ensures efficient recycling of 13C-labeled glycerol without de novo synthesis.
Enzymatic and Chemoenzymatic Approaches
Enzymatic synthesis of this compound leverages the specificity of glycerol kinase and glycerol-3-phosphate dehydrogenase to incorporate 13C into the glycerol backbone. In hepatic studies, [U-13C3]glycerol is phosphorylated to glycerol-3-phosphate, which enters gluconeogenesis or glycine synthesis pathways .
Gluconeogenic Flux Analysis
Isotopomer analysis of glucose derived from [U-13C3]glycerol reveals triple-labeled species (e.g., [1,2,3–13C3]glucose), confirming the retention of 13C during gluconeogenesis . Concurrently, 13C-labeled dihydroxyacetone phosphate (DHAP) and glyceraldehyde-3-phosphate (GA3P) serve as intermediates, with ∼30% of hepatic this compound flux directed toward glutathione synthesis via the serine-glycine pathway .
Chemoenzymatic Labeling in Pharmaceuticals
In drug formulations, this compound is synthesized via lipase-catalyzed transesterification of 13C-enriched triglycerides. This method ensures high enantiomeric purity and avoids racemization, critical for injectable formulations where glycerol serves as an excipient . The process is monitored using 13C NMR, with δ 62.5 (C1/C3) and δ 73.1 (C2) serving as quality control markers .
Challenges and Methodological Considerations
Signal Overlap in NMR Spectra
While 13C NMR reduces signal overlap compared to 1H NMR, the methine carbon of this compound (δ 73.1) may still co-elute with carbohydrate signals in complex mixtures . Employing heteronuclear single quantum coherence (HSQC) spectroscopy resolves this by correlating 13C and 1H shifts, enhancing specificity for this compound detection .
Metabolic Dilution in Biological Systems
In vivo labeling efficiency is hampered by endogenous unlabeled glycerol pools. Studies in C. reinhardtii show that only 33% of TAG-associated glycerol originates from recycled membrane lipids, necessitating high precursor concentrations (≥5 mM [13C2]acetate) to achieve detectable enrichment .
Cost and Scalability
Chemical synthesis of this compound remains cost-prohibitive for large-scale applications due to the expense of 13C precursors. Metabolic labeling in algae offers a scalable alternative, with yields of ∼14 mg/L culture under optimized nitrogen-deprivation conditions .
化学反応の分析
Types of Reactions
Glycerol-13C undergoes various chemical reactions, including:
Oxidation: It can be oxidized to form carbon-13 labeled dihydroxyacetone or glyceric acid.
Reduction: It can be reduced to form carbon-13 labeled propane-1,2-diol.
Substitution: It can undergo substitution reactions to form various derivatives.
Common Reagents and Conditions
Oxidation: Common oxidizing agents include potassium permanganate and hydrogen peroxide.
Reduction: Sodium borohydride and lithium aluminum hydride are commonly used reducing agents.
Substitution: Reagents such as acyl chlorides and alkyl halides are used for substitution reactions.
Major Products Formed
Oxidation: Carbon-13 labeled dihydroxyacetone and glyceric acid.
Reduction: Carbon-13 labeled propane-1,2-diol.
Substitution: Various carbon-13 labeled esters and ethers.
科学的研究の応用
Metabolic Studies
Glycerol as a Tracer in Metabolic Flux Analysis
Glycerol-13C is widely used in metabolic flux analysis (MFA) to trace metabolic pathways and identify biochemical processes. For instance, studies have demonstrated that this compound can elucidate the metabolic pathways in Mycobacterium tuberculosis. Using 13C-labeled glycerol, researchers identified an unusual carbon fixation pathway involving the glyoxylate shunt and anaplerotic reactions, which are crucial for understanding lipid metabolism in this bacterium .
Case Study: Gluconeogenesis Tracking
An important application of this compound is in tracking gluconeogenesis. A study involving healthy human subjects analyzed the effects of an oral load of [U-13C3]glycerol on glucose production. The results indicated that glycerol is a significant gluconeogenic precursor, with 13C enrichment in triglycerides (TAGs) being sensitive to nutritional states. The study found that under fasting conditions, 13C enrichment peaked rapidly, demonstrating glycerol's role in energy metabolism .
Nuclear Magnetic Resonance (NMR) Spectroscopy
Quantitative Analysis Using NMR
This compound is instrumental in NMR spectroscopy for quantitative analysis of glycerol in various samples, including pharmaceuticals. High-resolution 1H and 13C NMR methods have been validated for determining glycerol concentrations without complex sample preparation. These methods showed high specificity and accuracy, making them suitable for analyzing drug injections where glycerol content must be quantified .
Table 1: NMR Method Validation Results
Parameter | 1H NMR | 13C NMR |
---|---|---|
RSD (Repeatability) | 0.55% | 1.48% |
RSD (Stability) | 0.35% | 0.96% |
Average Recovery | 95.8% | 101.8% |
LOD (Limit of Detection) | 0.015 mM | 0.16 mM |
LOQ (Limit of Quantification) | 0.045 mM | 0.48 mM |
Protein Structure Determination
Isotopic Labeling for Structural Studies
This compound is also utilized in protein NMR studies to enhance the resolution of spectral data. By labeling proteins with either 1,3-13C or 2-13C glycerol, researchers can reduce spectral overlap and improve the clarity of structural information obtained from solid-state magic angle spinning (MAS) NMR experiments. This labeling technique allows for better assignment of amino acids and facilitates the determination of protein structures .
Advantages of this compound Labeling
- Reduced J-Couplings: The labeling minimizes J-couplings between neighboring atoms, leading to sharper spectral lines.
- Decreased Spectral Overlap: Enhanced clarity allows for more accurate structural assignments.
- Long-range Correlations: Weak dipolar couplings can be observed more easily due to reduced strong couplings.
作用機序
The mechanism of action of Glycerol-13C involves its incorporation into metabolic pathways where it can be detected using NMR spectroscopy. The carbon-13 isotope acts as a tracer, allowing researchers to monitor the transformation and distribution of the compound in various biological and chemical systems. The molecular targets and pathways involved depend on the specific application and the biological system being studied .
類似化合物との比較
Comparison with Similar 13C-Labeled Compounds
To contextualize glycerol-13C’s utility, a comparative analysis with structurally or functionally related 13C-labeled compounds is essential. Key examples include glycerol carbonate-(carbonyl-13C) , glycine-13C , and glucose-13C .
Table 1: Comparative Properties of this compound and Related Compounds
Structural and Functional Differences
- Glycerol Derivatives : this compound and glycerol carbonate-13C share a glycerol backbone but differ in functional groups. Glycerol carbonate-13C’s cyclic carbonate structure alters its chemical reactivity, making it suitable for polymer synthesis rather than metabolic studies .
- Amino Acids: Glycine-13C variants (e.g., glycine-1-13C vs. glycine-2-13C) demonstrate how labeling position affects metabolic incorporation. Glycine-2-13C is preferentially used in studies of serine synthesis via the glycine cleavage system .
- Carbohydrates: Glucose-13C and this compound both serve as tracers in energy metabolism but diverge in enrichment patterns. Glucose-13C oral administration yields higher systemic enrichment due to intestinal absorption efficiency, whereas this compound is more efficiently utilized via intravenous delivery .
Metabolic and Analytical Performance
- Enrichment Efficiency: this compound shows higher systemic enrichment (29.47%) with intravenous administration compared to oral routes, contrasting with glucose-13C, which favors oral uptake .
生物活性
Glycerol-13C, specifically labeled as [U-13C3]glycerol, is a stable isotope-labeled compound widely utilized in metabolic studies to probe various biochemical pathways in living organisms. Its unique labeling allows researchers to trace metabolic processes in real-time, particularly in human liver metabolism. This article delves into the biological activity of this compound, highlighting key research findings, case studies, and methodological advancements.
Overview of this compound
Glycerol is a simple polyol compound that serves as a backbone for triglycerides and phospholipids. The incorporation of carbon-13 isotope allows for enhanced tracking of glycerol metabolism through nuclear magnetic resonance (NMR) spectroscopy and other analytical techniques. This compound is particularly valuable for studying metabolic pathways such as gluconeogenesis, fatty acid synthesis, and the pentose phosphate pathway.
Key Metabolic Pathways Involving this compound
- Gluconeogenesis : this compound is primarily converted into glucose through gluconeogenesis. Studies have shown that the metabolic conversion of [U-13C3]glycerol leads to the formation of glucose isotopomers, indicating active gluconeogenic processes in the liver. For instance, one study demonstrated that under fasting conditions, glycerol contributes significantly to glucose production via the tricarboxylic acid (TCA) cycle .
- Fatty Acid Esterification : this compound also plays a crucial role in the synthesis of triacylglycerols (TAGs). The incorporation of 13C-labeled glycerol into TAGs provides insights into lipid metabolism under different nutritional states. Research indicates that fatty acid esterification is influenced by dietary intake; it is transient during fasting but continuous when fed .
- Pentose Phosphate Pathway : The pentose phosphate pathway (PPP) is another critical metabolic route where this compound is involved. The activity of this pathway can be assessed through NMR analysis of blood metabolites, providing biomarkers for mitochondrial function in hepatic tissues .
Case Study 1: Human Metabolism of this compound
A pivotal study conducted on human subjects examined the effects of an oral load of [U-13C3]glycerol on metabolic processes. Blood samples were analyzed using high-resolution NMR spectroscopy at various time points post-ingestion. The results indicated that:
- Peak 13C Enrichment : Maximum enrichment in TAG glycerol backbones was observed at approximately 90 minutes after ingestion during fasting conditions, with a peak enrichment of 7.1% .
- Nutritional State Influence : The rate of glycerol metabolism varied significantly between fasting and fed states, highlighting the dynamic nature of hepatic metabolism .
Case Study 2: Metabolic Profiling in Trichomonas vaginalis
Another study utilized 13C-NMR to investigate glycerol metabolism in the protozoan parasite Trichomonas vaginalis. This research uncovered glycerol as a major metabolite, indicating its importance not only in human metabolism but also in parasitic energy metabolism .
Methodological Advances
Recent advancements in NMR spectroscopy have enhanced the specificity and sensitivity of glycerol analysis:
- High-resolution NMR Techniques : These techniques allow for precise quantification and differentiation between glycerol and other metabolites in complex mixtures, improving the accuracy of metabolic assessments .
- Quantitative Analysis : Validation studies have demonstrated high repeatability and recovery rates for glycerol measurements using both 1H and 13C NMR methods, making them reliable tools for biological research .
Q & A
Basic Research Questions
Q. What are the established methodologies for synthesizing glycerol-<sup>13</sup>C, and how do researchers ensure isotopic purity?
Glycerol-<sup>13</sup>C is synthesized via photosynthetic pathways in organisms like cyanobacteria or algae, where <sup>13</sup>C-labeled carbon sources are metabolically incorporated . For chemical synthesis, protocols often involve <sup>13</sup>C-enriched precursors (e.g., <sup>13</sup>C-labeled glycerol carbonate) and catalytic processes to minimize isotopic dilution . Key steps include:
- Isotopic purity validation : Using nuclear magnetic resonance (NMR) or mass spectrometry (MS) to confirm ≥99 atom% <sup>13</sup>C enrichment .
- Purification : Chromatographic techniques (e.g., HPLC) to remove unlabeled byproducts .
Table 1 : Isotopic Purity of Commercial Glycerol-<sup>13</sup>C Products
Product ID | Isotopic Purity | Chemical Purity |
---|---|---|
930148 | ≥99 atom% <sup>13</sup>C | ≥99% (CP) |
802492 | ≥99 atom% <sup>13</sup>C | ≥95% (CP) |
Q. How is glycerol-<sup>13</sup>C utilized in NMR-based metabolic studies, and what parameters affect data accuracy?
Glycerol-<sup>13</sup>C is used to track metabolic flux in biological systems, such as cyanobacterial osmoregulation . NMR parameters critical for data reliability include:
- Relaxation times (T1) : Affected by radical concentrations (e.g., Finland trityl radicals at 5–20 mM reduce T1 by 40–60%) .
- Decoupling methods : Proton decoupling improves <sup>13</sup>C signal resolution .
Table 2 : T1 Relaxation Times of Glycerol-<sup>13</sup>C Under Different Conditions
Radical Concentration (mM) | T1 (s) | H-Decoupling |
---|---|---|
0 | 12.5 | No |
10 | 7.2 | Yes |
Advanced Research Questions
Q. How can researchers resolve contradictions in metabolic flux data derived from glycerol-<sup>13</sup>C tracing experiments?
Discrepancies often arise from isotopic dilution, compartmentalized metabolism, or analytical limitations. Mitigation strategies include:
- Compartmental modeling : Accounting for subcellular <sup>13</sup>C distribution in eukaryotic systems .
- Cross-validation : Pairing NMR with MS to compare isotopic enrichment patterns .
- Controlled experimental design : Standardizing culture conditions (e.g., light intensity, carbon source availability) to reduce biological variability .
Q. What advanced techniques optimize the use of glycerol-<sup>13</sup>C in dynamic nuclear polarization (DNP) studies?
DNP enhances NMR sensitivity by transferring polarization from electrons to <sup>13</sup>C nuclei. For glycerol-<sup>13</sup>C:
- Radical selection : Finland trityl radicals at 10 mM increase polarization efficiency by 3-fold compared to lower concentrations .
- Temperature control : Experiments conducted at 1.2 K to prolong hyperpolarized state stability .
- Pulse sequence optimization : Inversion recovery sequences with proton decoupling improve signal-to-noise ratios .
Q. How do researchers validate the incorporation of glycerol-<sup>13</sup>C into complex biological systems, such as lipid membranes?
Methodological approaches include:
- Isotopic profiling : Extracting lipids post-experiment and analyzing <sup>13</sup>C enrichment via gas chromatography-MS (GC-MS) .
- Kinetic modeling : Fitting time-resolved <sup>13</sup>C NMR data to estimate turnover rates of glycerol-derived lipids .
- Control experiments : Using unlabeled glycerol to distinguish background signals from labeled metabolic products .
Q. Methodological Best Practices
- Literature review : Use Google Scholar advanced operators (e.g.,
"glycerol-13C" AND ("NMR" OR "mass spectrometry")
) to identify seminal studies and track citations for emerging techniques . - Data reporting : Follow IMRaD structure (Introduction, Methods, Results, and Discussion) to ensure reproducibility, with detailed protocols for synthesis and validation .
- Ethical compliance : Disclose financial conflicts and adhere to data protection regulations when handling human or animal-derived samples .
特性
IUPAC Name |
(113C)propane-1,2,3-triol | |
---|---|---|
Source | PubChem | |
URL | https://pubchem.ncbi.nlm.nih.gov | |
Description | Data deposited in or computed by PubChem | |
InChI |
InChI=1S/C3H8O3/c4-1-3(6)2-5/h3-6H,1-2H2/i1+1 | |
Source | PubChem | |
URL | https://pubchem.ncbi.nlm.nih.gov | |
Description | Data deposited in or computed by PubChem | |
InChI Key |
PEDCQBHIVMGVHV-OUBTZVSYSA-N | |
Source | PubChem | |
URL | https://pubchem.ncbi.nlm.nih.gov | |
Description | Data deposited in or computed by PubChem | |
Canonical SMILES |
C(C(CO)O)O | |
Source | PubChem | |
URL | https://pubchem.ncbi.nlm.nih.gov | |
Description | Data deposited in or computed by PubChem | |
Isomeric SMILES |
C(C([13CH2]O)O)O | |
Source | PubChem | |
URL | https://pubchem.ncbi.nlm.nih.gov | |
Description | Data deposited in or computed by PubChem | |
Molecular Formula |
C3H8O3 | |
Source | PubChem | |
URL | https://pubchem.ncbi.nlm.nih.gov | |
Description | Data deposited in or computed by PubChem | |
Molecular Weight |
93.09 g/mol | |
Source | PubChem | |
URL | https://pubchem.ncbi.nlm.nih.gov | |
Description | Data deposited in or computed by PubChem | |
試験管内研究製品の免責事項と情報
BenchChemで提示されるすべての記事および製品情報は、情報提供を目的としています。BenchChemで購入可能な製品は、生体外研究のために特別に設計されています。生体外研究は、ラテン語の "in glass" に由来し、生物体の外で行われる実験を指します。これらの製品は医薬品または薬として分類されておらず、FDAから任何の医療状態、病気、または疾患の予防、治療、または治癒のために承認されていません。これらの製品を人間または動物に体内に導入する形態は、法律により厳格に禁止されています。これらのガイドラインに従うことは、研究と実験において法的および倫理的な基準の遵守を確実にするために重要です。