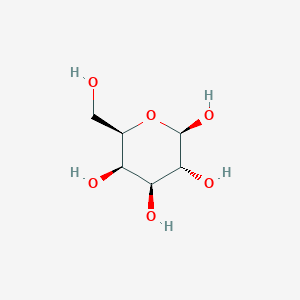
beta-D-galactose
概要
説明
Beta-D-Galactose, also known as b-D-galactose or beta-D-gal, belongs to the class of organic compounds known as hexoses. These are monosaccharides in which the sugar unit is a is a six-carbon containing moeity. This compound is soluble (in water) and a very weakly acidic compound (based on its pKa). This compound has been found throughout most human tissues, and has also been primarily detected in feces. Within the cell, this compound is primarily located in the lysosome and myelin sheath. This compound exists in all eukaryotes, ranging from yeast to humans. This compound can be converted into D-galactose through its interaction with the enzyme galactose mutarotase.
This compound is a D-galactopyranose having beta-configuration at the anomeric centre. It has a role as an epitope and a mouse metabolite. It is an enantiomer of a beta-L-galactose.
作用機序
Target of Action
Beta-D-Galactose primarily targets the enzyme beta-D-galactosidase (BGAL) . BGAL is a hydrolytic enzyme that catalyzes the breakdown of lactose into glucose and galactose . It targets xyloglucans, the most abundant type of hemicellulose in the primary cell wall of all vascular plants . BGAL is associated with cell wall biogenesis and modification .
Mode of Action
The catalytic process of BGAL involves the transfer of a sugar residue from a glycosyl donor to an acceptor via a double-displacement mechanism . When water acts as an acceptor, hydrolysis prevails, resulting in the production of lactose-free products . When lactose acts as an acceptor, transgalactosylation prevails, resulting in the production of prebiotic oligosaccharides .
Biochemical Pathways
This compound is primarily metabolized via the Leloir pathway . This pathway consists of four enzymes: galactose mutarotase (GALM), galactokinase (GALK1), galactose-1-phosphate uridylyltransferase (GALT), and UDP-galactose-4’-epimerase (GALE) . The conversion of this compound to glucose 1-phosphate is accomplished by the action of these enzymes .
Pharmacokinetics
The pharmacokinetics of this compound is influenced by its formulation. For instance, when formulated into nanoparticles using polydopamine as a drug carrier, the bioavailability and liver accumulation of this compound were significantly enhanced .
Result of Action
The hydrolysis of lactose to glucose and galactose by BGAL is an important process in the food industry because of the potentially beneficial effects of assimilating food containing lactose . The hydrolytic and transgalactosylation properties of BGAL have several advantages in the food and dairy industries . Some of the benefits include the production of lactose-free products for lactose-intolerant people .
Action Environment
The activity of this compound can be influenced by environmental factors. For instance, psychrophilic Beta-D-Galactosidases, which are adapted to cold environments, have been found to have a stable structure at refrigerated temperatures . This allows for more effective biochemical pathways with shorter reaction times, less energy usage, and ecological acceptability .
生化学分析
Biochemical Properties
Beta-D-Galactose interacts with several enzymes, proteins, and other biomolecules. One of the most significant enzymes it interacts with is Beta-Galactosidase, a glycoside hydrolase enzyme. This enzyme catalyzes the hydrolysis of this compound from lactose, breaking the glycosidic bond and resulting in the production of glucose and galactose . The interaction between this compound and Beta-Galactosidase is a key biochemical reaction that has significant implications in the food and dairy industries .
Cellular Effects
This compound has profound effects on various types of cells and cellular processes. It influences cell function by participating in cell signaling pathways and affecting gene expression. For instance, in lactose-intolerant individuals, the absence of Beta-Galactosidase in the small intestine leads to undigested this compound reaching the large intestine, where it is fermented by colonic microorganisms. This fermentation process can cause abdominal pain, diarrhea, and nausea .
Molecular Mechanism
The molecular mechanism of this compound involves its binding interactions with biomolecules, enzyme inhibition or activation, and changes in gene expression. The catalytic process of Beta-Galactosidase involves the transfer of a sugar residue from a glycosyl donor (like lactose) to an acceptor (like water or another lactose molecule) via a double-displacement mechanism .
Metabolic Pathways
This compound is involved in several metabolic pathways. It interacts with various enzymes and cofactors, and can affect metabolic flux or metabolite levels. For instance, it is a key player in the Leloir pathway, a metabolic pathway for the catabolism of this compound to glucose-1-phosphate .
Subcellular Localization
It is known that this compound can be found in various compartments or organelles within the cell due to its involvement in numerous metabolic pathways
生物活性
Beta-D-galactose, a monosaccharide and a component of lactose, plays significant roles in various biological processes. Its biological activity is largely influenced by its structural characteristics and interactions with other biomolecules. This article provides an overview of the biological activities of this compound, including its effects on aging, oxidative stress, and cellular functions, supported by relevant research findings and case studies.
Chemical Structure and Properties
This compound is an aldohexose sugar that can exist in both pyranose and furanose forms. It is a reducing sugar, meaning it can act as a reducing agent in biochemical reactions. This property is crucial for its role in glycosylation processes, where it contributes to the formation of glycoproteins and glycolipids.
Biological Functions
1. Role in Aging and Oxidative Stress
Research has shown that this compound is often used to induce aging in animal models. The administration of D-galactose has been linked to increased oxidative stress, which accelerates aging processes. For instance, a study demonstrated that Wistar rats treated with D-galactose exhibited elevated levels of oxidative stress markers such as malondialdehyde (MDA) and 8-hydroxy-2'-deoxyguanosine (8-OHdG), indicating significant oxidative damage to DNA and lipids .
2. Effects on Cardiovascular Health
D-galactose-induced aging models have also been utilized to study cardiovascular health. In neonatal rat cardiomyocytes, exposure to D-galactose resulted in increased senescence markers and protein oxidation levels, suggesting that this compound plays a role in cardiac aging . The following table summarizes key findings from various studies on the effects of D-galactose on cardiac health:
Study Model | Age | D-Galactose Dose (mg/kg/day) | Route | Duration | Major Findings |
---|---|---|---|---|---|
Wistar rats | 5 months | 60 | IP injection | 6 weeks | Increased AGE protein levels; cardiac senescence observed |
SD rats | 3 months | 400 | IP injection | 6 weeks | Increased oxidative stress markers; reduced antioxidants |
C57BL/6J mice | 2 months | 50 | SC injection | 8 weeks | Activation of p53-p21 signaling pathway |
Immunological Effects
This compound has been implicated in modulating immune responses. Studies indicate that it can influence the expression of beta-galactosidase, an enzyme involved in the metabolism of galactose-containing compounds. Elevated beta-galactosidase activity has been observed in tissues subjected to oxidative stress induced by D-galactose treatment, suggesting a potential mechanism for cellular response to injury .
Case Studies
Case Study: D-Galactose-Induced Aging in Rats
A notable study investigated the effects of D-galactose on bone health in male Wistar rats subjected to high-fat diets. The results indicated that D-galactose exacerbated bone dyshomeostasis and inflammation over time, highlighting its role in age-related metabolic disorders . The findings suggest that interventions targeting oxidative stress could mitigate some adverse effects associated with D-galactose-induced aging.
Case Study: Neuroprotective Effects
Another research effort focused on neuroprotective strategies against D-galactose-induced oxidative stress in rat models. Diosgenin was found to alleviate oxidative injuries caused by D-galactose treatment, indicating potential therapeutic avenues for conditions linked to oxidative damage .
科学的研究の応用
Food Industry Applications
Lactose Hydrolysis
Beta-D-galactose is primarily known for its role in the hydrolysis of lactose through the enzyme beta-galactosidase. This process is essential for producing lactose-free dairy products, catering to lactose-intolerant individuals. The hydrolysis reduces lactose content, preventing crystallization in frozen and concentrated desserts, thereby improving texture and taste .
Whey Treatment
The dairy industry generates a significant amount of whey as a byproduct. Beta-galactosidase is employed to treat whey, converting lactose into this compound and thus addressing environmental concerns related to whey disposal. This treatment not only minimizes waste but also enhances the nutritional profile of the whey .
Application | Description | Benefits |
---|---|---|
Lactose Hydrolysis | Conversion of lactose to this compound | Produces lactose-free products |
Whey Treatment | Hydrolysis of whey to reduce environmental impact | Nutritional enhancement and waste reduction |
Biotechnology Applications
Enzyme-Linked Immunosorbent Assays (ELISAs)
Beta-galactosidase serves as a biomarker in various diagnostic applications, including ELISAs. Its activity can be measured to assess enzyme levels in biological samples, aiding in disease diagnosis and monitoring .
Gene Expression Studies
In molecular biology, beta-galactosidase is utilized as a reporter gene in gene expression studies. Its expression can be easily monitored through colorimetric assays, facilitating the understanding of gene regulation mechanisms .
Fluorescent Probes for Imaging
Recent advancements have led to the development of fluorescent probes that utilize beta-galactosidase for cellular imaging. These probes allow for the detection of beta-galactosidase activity in live cells, providing insights into cellular processes such as senescence and cancer progression .
Pharmaceutical Applications
Drug Delivery Systems
this compound has been investigated as a scaffold for drug delivery systems due to its biocompatibility and ability to enhance drug solubility. It can be conjugated with therapeutic agents to improve their pharmacokinetics and target specific tissues or cells .
Theranostics
In theranostic applications (therapeutic + diagnostic), this compound derivatives are being explored for their potential in targeted therapy and imaging in cancer treatment. These compounds can deliver drugs directly to cancer cells while allowing for simultaneous imaging .
Case Studies
Case Study 1: Lactose-Free Dairy Products
A study conducted by Karasova et al. (2002) demonstrated that using beta-galactosidase from Bifidobacterium species effectively reduced lactose content in milk, making it suitable for lactose-intolerant consumers. The research highlighted the enzyme's efficiency in hydrolyzing lactose without compromising the nutritional quality of the milk .
Case Study 2: Whey Protein Recovery
Research by Domingues et al. (2005) focused on recovering high-functioning whey proteins through ultrafiltration combined with beta-galactosidase treatment. The study found that this approach not only enhanced protein recovery but also reduced biochemical oxygen demand (BOD), addressing environmental concerns associated with whey disposal .
Case Study | Focus Area | Findings |
---|---|---|
Lactose-Free Dairy | Hydrolysis efficiency | Effective reduction of lactose content |
Whey Protein Recovery | Environmental impact | Enhanced protein recovery and reduced BOD |
特性
IUPAC Name |
(2R,3R,4S,5R,6R)-6-(hydroxymethyl)oxane-2,3,4,5-tetrol | |
---|---|---|
Source | PubChem | |
URL | https://pubchem.ncbi.nlm.nih.gov | |
Description | Data deposited in or computed by PubChem | |
InChI |
InChI=1S/C6H12O6/c7-1-2-3(8)4(9)5(10)6(11)12-2/h2-11H,1H2/t2-,3+,4+,5-,6-/m1/s1 | |
Source | PubChem | |
URL | https://pubchem.ncbi.nlm.nih.gov | |
Description | Data deposited in or computed by PubChem | |
InChI Key |
WQZGKKKJIJFFOK-FPRJBGLDSA-N | |
Source | PubChem | |
URL | https://pubchem.ncbi.nlm.nih.gov | |
Description | Data deposited in or computed by PubChem | |
Canonical SMILES |
C(C1C(C(C(C(O1)O)O)O)O)O | |
Source | PubChem | |
URL | https://pubchem.ncbi.nlm.nih.gov | |
Description | Data deposited in or computed by PubChem | |
Isomeric SMILES |
C([C@@H]1[C@@H]([C@@H]([C@H]([C@@H](O1)O)O)O)O)O | |
Source | PubChem | |
URL | https://pubchem.ncbi.nlm.nih.gov | |
Description | Data deposited in or computed by PubChem | |
Molecular Formula |
C6H12O6 | |
Source | PubChem | |
URL | https://pubchem.ncbi.nlm.nih.gov | |
Description | Data deposited in or computed by PubChem | |
DSSTOX Substance ID |
DTXSID001015884 | |
Record name | beta-D-Galactopyranose | |
Source | EPA DSSTox | |
URL | https://comptox.epa.gov/dashboard/DTXSID001015884 | |
Description | DSSTox provides a high quality public chemistry resource for supporting improved predictive toxicology. | |
Molecular Weight |
180.16 g/mol | |
Source | PubChem | |
URL | https://pubchem.ncbi.nlm.nih.gov | |
Description | Data deposited in or computed by PubChem | |
Physical Description |
Solid | |
Record name | beta-D-Galactose | |
Source | Human Metabolome Database (HMDB) | |
URL | http://www.hmdb.ca/metabolites/HMDB0003449 | |
Description | The Human Metabolome Database (HMDB) is a freely available electronic database containing detailed information about small molecule metabolites found in the human body. | |
Explanation | HMDB is offered to the public as a freely available resource. Use and re-distribution of the data, in whole or in part, for commercial purposes requires explicit permission of the authors and explicit acknowledgment of the source material (HMDB) and the original publication (see the HMDB citing page). We ask that users who download significant portions of the database cite the HMDB paper in any resulting publications. | |
CAS No. |
7296-64-2 | |
Record name | β-D-Galactopyranose | |
Source | CAS Common Chemistry | |
URL | https://commonchemistry.cas.org/detail?cas_rn=7296-64-2 | |
Description | CAS Common Chemistry is an open community resource for accessing chemical information. Nearly 500,000 chemical substances from CAS REGISTRY cover areas of community interest, including common and frequently regulated chemicals, and those relevant to high school and undergraduate chemistry classes. This chemical information, curated by our expert scientists, is provided in alignment with our mission as a division of the American Chemical Society. | |
Explanation | The data from CAS Common Chemistry is provided under a CC-BY-NC 4.0 license, unless otherwise stated. | |
Record name | beta-D-Galactose | |
Source | ChemIDplus | |
URL | https://pubchem.ncbi.nlm.nih.gov/substance/?source=chemidplus&sourceid=0007296642 | |
Description | ChemIDplus is a free, web search system that provides access to the structure and nomenclature authority files used for the identification of chemical substances cited in National Library of Medicine (NLM) databases, including the TOXNET system. | |
Record name | beta-D-Galactopyranose | |
Source | EPA DSSTox | |
URL | https://comptox.epa.gov/dashboard/DTXSID001015884 | |
Description | DSSTox provides a high quality public chemistry resource for supporting improved predictive toxicology. | |
Record name | β-D-Galactopyranose | |
Source | FDA Global Substance Registration System (GSRS) | |
URL | https://gsrs.ncats.nih.gov/ginas/app/beta/substances/RQ6K52J67A | |
Description | The FDA Global Substance Registration System (GSRS) enables the efficient and accurate exchange of information on what substances are in regulated products. Instead of relying on names, which vary across regulatory domains, countries, and regions, the GSRS knowledge base makes it possible for substances to be defined by standardized, scientific descriptions. | |
Explanation | Unless otherwise noted, the contents of the FDA website (www.fda.gov), both text and graphics, are not copyrighted. They are in the public domain and may be republished, reprinted and otherwise used freely by anyone without the need to obtain permission from FDA. Credit to the U.S. Food and Drug Administration as the source is appreciated but not required. | |
Record name | beta-D-Galactose | |
Source | Human Metabolome Database (HMDB) | |
URL | http://www.hmdb.ca/metabolites/HMDB0003449 | |
Description | The Human Metabolome Database (HMDB) is a freely available electronic database containing detailed information about small molecule metabolites found in the human body. | |
Explanation | HMDB is offered to the public as a freely available resource. Use and re-distribution of the data, in whole or in part, for commercial purposes requires explicit permission of the authors and explicit acknowledgment of the source material (HMDB) and the original publication (see the HMDB citing page). We ask that users who download significant portions of the database cite the HMDB paper in any resulting publications. | |
Synthesis routes and methods I
Procedure details
Synthesis routes and methods II
Procedure details
Retrosynthesis Analysis
AI-Powered Synthesis Planning: Our tool employs the Template_relevance Pistachio, Template_relevance Bkms_metabolic, Template_relevance Pistachio_ringbreaker, Template_relevance Reaxys, Template_relevance Reaxys_biocatalysis model, leveraging a vast database of chemical reactions to predict feasible synthetic routes.
One-Step Synthesis Focus: Specifically designed for one-step synthesis, it provides concise and direct routes for your target compounds, streamlining the synthesis process.
Accurate Predictions: Utilizing the extensive PISTACHIO, BKMS_METABOLIC, PISTACHIO_RINGBREAKER, REAXYS, REAXYS_BIOCATALYSIS database, our tool offers high-accuracy predictions, reflecting the latest in chemical research and data.
Strategy Settings
Precursor scoring | Relevance Heuristic |
---|---|
Min. plausibility | 0.01 |
Model | Template_relevance |
Template Set | Pistachio/Bkms_metabolic/Pistachio_ringbreaker/Reaxys/Reaxys_biocatalysis |
Top-N result to add to graph | 6 |
Feasible Synthetic Routes
- A: Beta-D-galactose serves as a specific binding target for various lectins, both naturally occurring and those used as research tools [, , , , , , ]. For instance, Ricinus communis agglutinin I (RCA I) exhibits a high affinity for this compound residues [, , , , ]. This interaction allows researchers to investigate the distribution and changes in glycoconjugates containing this compound within cells and tissues. Furthermore, lectin binding to this compound-containing glycoproteins on cell surfaces can trigger various cellular responses, including agglutination and signaling cascades.
A: Calcium ions (Ca2+) play a crucial role in mediating the interaction between this compound and the sialic acid residues on HBP []. The presence of Ca2+ facilitates a stable binding conformation, influencing how HBP recognizes and binds to other molecules, a process vital for various physiological functions.
- A: While the provided research papers do not delve into detailed spectroscopic analysis, techniques like Nuclear Magnetic Resonance (NMR) spectroscopy can be employed to characterize the structure of this compound [, ]. NMR provides information about the arrangement of atoms within the molecule and can differentiate between different anomers of sugars, including alpha and beta forms.
- A: this compound serves as a substrate for the enzyme beta-galactosidase []. This enzyme specifically cleaves the glycosidic bond in Beta-D-galactosides. This specificity is exploited in enzymatic assays to measure lactose content []. The enzyme hydrolyzes lactose into D-glucose and D-galactose, and by measuring the amount of D-galactose produced, one can determine the initial lactose concentration.
- A: While not extensively detailed, the research hints at the application of computational chemistry in understanding this compound's conformational behavior []. For instance, studying torsional anharmonicity in this compound using methods like torsional path integral Monte Carlo simulations can provide insights into its conformational preferences and how these might influence its interactions with other molecules.
- A: The specific orientation of hydroxyl groups on the this compound molecule is crucial for its recognition by lectins and enzymes [, , , , ]. Modifications such as sulfation at specific positions (e.g., 3-O-sulfo-Beta-D-galactosylceramide) can alter its binding affinity and selectivity for certain antibodies and lectins []. Similarly, the presence or absence of acetyl groups, as seen in the comparison of this compound and N-acetyl-D-galactosamine recognition by lectins [, , , , ], highlights how subtle structural changes significantly influence its biological activity.
- A: this compound research exemplifies interdisciplinary synergy. Its study draws upon and contributes to:
試験管内研究製品の免責事項と情報
BenchChemで提示されるすべての記事および製品情報は、情報提供を目的としています。BenchChemで購入可能な製品は、生体外研究のために特別に設計されています。生体外研究は、ラテン語の "in glass" に由来し、生物体の外で行われる実験を指します。これらの製品は医薬品または薬として分類されておらず、FDAから任何の医療状態、病気、または疾患の予防、治療、または治癒のために承認されていません。これらの製品を人間または動物に体内に導入する形態は、法律により厳格に禁止されています。これらのガイドラインに従うことは、研究と実験において法的および倫理的な基準の遵守を確実にするために重要です。