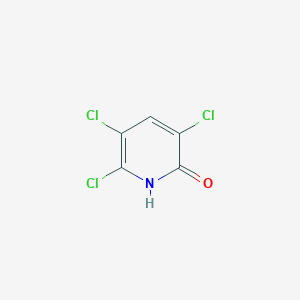
3,5,6-トリクロロ-2-ピリジノール
概要
説明
3,5,6-Trichloro-2-pyridinol is a chlorinated pyridine compound widely recognized for its role as a degradation product of the insecticide chlorpyrifos and the herbicide triclopyr . It is a highly toxic and persistent pollutant, often found in agricultural and industrial settings . This compound is known for its high solubility in water and long half-life, making it a significant environmental contaminant .
科学的研究の応用
3,5,6-Trichloro-2-pyridinol has several scientific research applications:
Chemistry: It is used as a model compound to study the degradation pathways of chlorinated pyridines.
Biology: Research on its biodegradation by microorganisms helps understand microbial detoxification processes.
Industry: It is used in the development of bioremediation strategies for contaminated soils and water bodies.
作用機序
Target of Action
3,5,6-Trichloro-2-pyridinol (TCP) is a metabolite of the insecticide chlorpyrifos and the herbicide triclopyr . It has been suggested that TCP has cytotoxic and endocrine-disrupting effects . The main target organ for chlorpyrifos, from which TCP is derived, is the nervous system .
Mode of Action
TCP has been found to be more toxic than chlorpyrifos for two mammalian cell lines (HEK293 and N2a) in in vitro assays . Both TCP and chlorpyrifos show a similar estrogenic activity, being between 2500 and 3000 times less estrogenic than 17β-estradiol .
Biochemical Pathways
The degradation of TCP has been studied with a strain of Micrococcus luteus, which was capable of degrading TCP . Two possible degradation pathways of TCP were proposed based on LC–MS analysis: the hydrolytic-oxidative dechlorination pathway and the denitrification pathway .
Pharmacokinetics
The pharmacokinetics of TCP has been evaluated in rat saliva . Experimental results suggest that TCP partitioning from plasma to saliva in rats is relatively constant over a range of varying physiological conditions . The TCP concentration in saliva was highly correlated to the amount of unbound TCP in plasma .
Result of Action
The cytotoxic and estrogenic activity of TCP has been evaluated by in vitro assays, using two mammalian cell lines (HEK293 and N2a), and a recombinant yeast . Results indicate that TCP is more toxic than chlorpyrifos for the two cell lines assayed, being N2a cells more sensitive to both compounds .
Action Environment
TCP is a highly toxic and refractory residue of both the insecticide chlorpyrifos and the herbicide triclopyr . Due to its high solubility in water and long half-life, TCP is listed as a mobile and persistent organic pollutant by the US Environmental Protection Agency . Moreover, TCP has a high antibacterial activity owing to the existence of three chloride atoms on the pyridinol ring .
生化学分析
Biochemical Properties
3,5,6-Trichloro-2-pyridinol is a metabolite of the insecticide chlorpyrifos and the herbicide triclopyr . It is produced by hydrolytic and photolytic mechanisms . The main mechanism of toxicity of 3,5,6-Trichloro-2-pyridinol is the inhibition of acetylcholinesterase .
Cellular Effects
3,5,6-Trichloro-2-pyridinol has been found to be toxic to a variety of aquatic organisms, including luminescent marine bacteria Aliivibrio fischeri, freshwater unicellular alga Pseudokirchneriella subcapitata, and cladoceran Daphnia magna . It has been observed that the mixture of 3,5,6-Trichloro-2-pyridinol and its parent compound chlorpyrifos is more toxic than the compounds tested separately .
Molecular Mechanism
The main mechanism of toxicity of 3,5,6-Trichloro-2-pyridinol is the inhibition of acetylcholinesterase . This enzyme is present in all vertebrates and its inhibition can affect non-target species .
Temporal Effects in Laboratory Settings
The degradation of 3,5,6-Trichloro-2-pyridinol has been studied with a novel strain Micrococcus luteus ML isolated from a stable TCP degrading microbiota . Strain ML was capable of degrading 61.6% of 3,5,6-Trichloro-2-pyridinol (50 mg/L) and 35.4% of chlorpyrifos (50 mg/L) at 24 h and 48 h under the optimal conditions .
Metabolic Pathways
The degradation of 3,5,6-Trichloro-2-pyridinol involves two possible degradation pathways based on LC–MS analysis . Both the hydrolytic-oxidative dechlorination pathway and the denitrification pathway might be involved in 3,5,6-Trichloro-2-pyridinol biodegradation .
Transport and Distribution
3,5,6-Trichloro-2-pyridinol is more mobile than the parent molecule due to its higher water solubility . This suggests that it can be transported and distributed within cells and tissues more easily than its parent compound.
準備方法
Synthetic Routes and Reaction Conditions
3,5,6-Trichloro-2-pyridinol can be synthesized through the hydrolysis of chlorpyrifos under acidic or basic conditions . The reaction typically involves the use of hydrochloric acid or sodium hydroxide as catalysts, resulting in the cleavage of the phosphorus-oxygen bond in chlorpyrifos, leading to the formation of 3,5,6-Trichloro-2-pyridinol .
Industrial Production Methods
Industrial production of 3,5,6-Trichloro-2-pyridinol primarily involves the large-scale hydrolysis of chlorpyrifos. This process is carried out in controlled environments to ensure the efficient conversion of chlorpyrifos to 3,5,6-Trichloro-2-pyridinol while minimizing the release of harmful by-products .
化学反応の分析
Types of Reactions
3,5,6-Trichloro-2-pyridinol undergoes various chemical reactions, including:
Oxidation: It can be oxidized to form chlorinated pyridine derivatives.
Reduction: Reduction reactions can lead to the formation of less chlorinated pyridine compounds.
Substitution: Nucleophilic substitution reactions can replace chlorine atoms with other functional groups.
Common Reagents and Conditions
Oxidation: Common oxidizing agents include potassium permanganate and hydrogen peroxide.
Reduction: Reducing agents such as sodium borohydride and lithium aluminum hydride are used.
Substitution: Nucleophiles like hydroxide ions and amines are commonly employed.
Major Products Formed
Oxidation: Chlorinated pyridine derivatives.
Reduction: Less chlorinated pyridine compounds.
Substitution: Various substituted pyridinol derivatives.
類似化合物との比較
3,5,6-Trichloro-2-pyridinol is unique due to its high toxicity and persistence compared to other chlorinated pyridines . Similar compounds include:
2,3,5-Trichloropyridine: Less toxic and less persistent.
3,5-Dichloro-2-pyridinol: Lower toxicity and shorter half-life.
2,4,6-Trichloropyridine: Different degradation pathways and environmental behavior.
特性
IUPAC Name |
3,5,6-trichloro-1H-pyridin-2-one | |
---|---|---|
Source | PubChem | |
URL | https://pubchem.ncbi.nlm.nih.gov | |
Description | Data deposited in or computed by PubChem | |
InChI |
InChI=1S/C5H2Cl3NO/c6-2-1-3(7)5(10)9-4(2)8/h1H,(H,9,10) | |
Source | PubChem | |
URL | https://pubchem.ncbi.nlm.nih.gov | |
Description | Data deposited in or computed by PubChem | |
InChI Key |
WCYYAQFQZQEUEN-UHFFFAOYSA-N | |
Source | PubChem | |
URL | https://pubchem.ncbi.nlm.nih.gov | |
Description | Data deposited in or computed by PubChem | |
Canonical SMILES |
C1=C(C(=O)NC(=C1Cl)Cl)Cl | |
Source | PubChem | |
URL | https://pubchem.ncbi.nlm.nih.gov | |
Description | Data deposited in or computed by PubChem | |
Molecular Formula |
C5H2Cl3NO | |
Source | PubChem | |
URL | https://pubchem.ncbi.nlm.nih.gov | |
Description | Data deposited in or computed by PubChem | |
Related CAS |
37439-34-2 (hydrochloride salt) | |
Record name | 3,5,6-Trichloro-2-pyridinol | |
Source | ChemIDplus | |
URL | https://pubchem.ncbi.nlm.nih.gov/substance/?source=chemidplus&sourceid=0006515384 | |
Description | ChemIDplus is a free, web search system that provides access to the structure and nomenclature authority files used for the identification of chemical substances cited in National Library of Medicine (NLM) databases, including the TOXNET system. | |
DSSTOX Substance ID |
DTXSID7038317 | |
Record name | 3,5,6-Trichloro-2-pyridinol | |
Source | EPA DSSTox | |
URL | https://comptox.epa.gov/dashboard/DTXSID7038317 | |
Description | DSSTox provides a high quality public chemistry resource for supporting improved predictive toxicology. | |
Molecular Weight |
198.43 g/mol | |
Source | PubChem | |
URL | https://pubchem.ncbi.nlm.nih.gov | |
Description | Data deposited in or computed by PubChem | |
Physical Description |
Water or Solvent Wet Solid, Solid | |
Record name | 2(1H)-Pyridinone, 3,5,6-trichloro- | |
Source | EPA Chemicals under the TSCA | |
URL | https://www.epa.gov/chemicals-under-tsca | |
Description | EPA Chemicals under the Toxic Substances Control Act (TSCA) collection contains information on chemicals and their regulations under TSCA, including non-confidential content from the TSCA Chemical Substance Inventory and Chemical Data Reporting. | |
Record name | 3,5,6-Trichloro-2-pyridinol | |
Source | Human Metabolome Database (HMDB) | |
URL | http://www.hmdb.ca/metabolites/HMDB0039853 | |
Description | The Human Metabolome Database (HMDB) is a freely available electronic database containing detailed information about small molecule metabolites found in the human body. | |
Explanation | HMDB is offered to the public as a freely available resource. Use and re-distribution of the data, in whole or in part, for commercial purposes requires explicit permission of the authors and explicit acknowledgment of the source material (HMDB) and the original publication (see the HMDB citing page). We ask that users who download significant portions of the database cite the HMDB paper in any resulting publications. | |
CAS No. |
6515-38-4 | |
Record name | 3,5,6-Trichloro-2-pyridinol | |
Source | CAS Common Chemistry | |
URL | https://commonchemistry.cas.org/detail?cas_rn=6515-38-4 | |
Description | CAS Common Chemistry is an open community resource for accessing chemical information. Nearly 500,000 chemical substances from CAS REGISTRY cover areas of community interest, including common and frequently regulated chemicals, and those relevant to high school and undergraduate chemistry classes. This chemical information, curated by our expert scientists, is provided in alignment with our mission as a division of the American Chemical Society. | |
Explanation | The data from CAS Common Chemistry is provided under a CC-BY-NC 4.0 license, unless otherwise stated. | |
Record name | 3,5,6-Trichloro-2-pyridinol | |
Source | ChemIDplus | |
URL | https://pubchem.ncbi.nlm.nih.gov/substance/?source=chemidplus&sourceid=0006515384 | |
Description | ChemIDplus is a free, web search system that provides access to the structure and nomenclature authority files used for the identification of chemical substances cited in National Library of Medicine (NLM) databases, including the TOXNET system. | |
Record name | 2(1H)-Pyridinone, 3,5,6-trichloro- | |
Source | EPA Chemicals under the TSCA | |
URL | https://www.epa.gov/chemicals-under-tsca | |
Description | EPA Chemicals under the Toxic Substances Control Act (TSCA) collection contains information on chemicals and their regulations under TSCA, including non-confidential content from the TSCA Chemical Substance Inventory and Chemical Data Reporting. | |
Record name | 3,5,6-Trichloro-2-pyridinol | |
Source | EPA DSSTox | |
URL | https://comptox.epa.gov/dashboard/DTXSID7038317 | |
Description | DSSTox provides a high quality public chemistry resource for supporting improved predictive toxicology. | |
Record name | 3,5,6-trichloro-2-pyridone | |
Source | European Chemicals Agency (ECHA) | |
URL | https://echa.europa.eu/substance-information/-/substanceinfo/100.026.733 | |
Description | The European Chemicals Agency (ECHA) is an agency of the European Union which is the driving force among regulatory authorities in implementing the EU's groundbreaking chemicals legislation for the benefit of human health and the environment as well as for innovation and competitiveness. | |
Explanation | Use of the information, documents and data from the ECHA website is subject to the terms and conditions of this Legal Notice, and subject to other binding limitations provided for under applicable law, the information, documents and data made available on the ECHA website may be reproduced, distributed and/or used, totally or in part, for non-commercial purposes provided that ECHA is acknowledged as the source: "Source: European Chemicals Agency, http://echa.europa.eu/". Such acknowledgement must be included in each copy of the material. ECHA permits and encourages organisations and individuals to create links to the ECHA website under the following cumulative conditions: Links can only be made to webpages that provide a link to the Legal Notice page. | |
Record name | 3,5,6-TRICHLORO-2-PYRIDINONE | |
Source | FDA Global Substance Registration System (GSRS) | |
URL | https://gsrs.ncats.nih.gov/ginas/app/beta/substances/JS52YZJ84A | |
Description | The FDA Global Substance Registration System (GSRS) enables the efficient and accurate exchange of information on what substances are in regulated products. Instead of relying on names, which vary across regulatory domains, countries, and regions, the GSRS knowledge base makes it possible for substances to be defined by standardized, scientific descriptions. | |
Explanation | Unless otherwise noted, the contents of the FDA website (www.fda.gov), both text and graphics, are not copyrighted. They are in the public domain and may be republished, reprinted and otherwise used freely by anyone without the need to obtain permission from FDA. Credit to the U.S. Food and Drug Administration as the source is appreciated but not required. | |
Record name | 3,5,6-Trichloro-2-pyridinol | |
Source | Human Metabolome Database (HMDB) | |
URL | http://www.hmdb.ca/metabolites/HMDB0039853 | |
Description | The Human Metabolome Database (HMDB) is a freely available electronic database containing detailed information about small molecule metabolites found in the human body. | |
Explanation | HMDB is offered to the public as a freely available resource. Use and re-distribution of the data, in whole or in part, for commercial purposes requires explicit permission of the authors and explicit acknowledgment of the source material (HMDB) and the original publication (see the HMDB citing page). We ask that users who download significant portions of the database cite the HMDB paper in any resulting publications. | |
Melting Point |
208 - 209 °C | |
Record name | 3,5,6-Trichloro-2-pyridinol | |
Source | Human Metabolome Database (HMDB) | |
URL | http://www.hmdb.ca/metabolites/HMDB0039853 | |
Description | The Human Metabolome Database (HMDB) is a freely available electronic database containing detailed information about small molecule metabolites found in the human body. | |
Explanation | HMDB is offered to the public as a freely available resource. Use and re-distribution of the data, in whole or in part, for commercial purposes requires explicit permission of the authors and explicit acknowledgment of the source material (HMDB) and the original publication (see the HMDB citing page). We ask that users who download significant portions of the database cite the HMDB paper in any resulting publications. | |
Retrosynthesis Analysis
AI-Powered Synthesis Planning: Our tool employs the Template_relevance Pistachio, Template_relevance Bkms_metabolic, Template_relevance Pistachio_ringbreaker, Template_relevance Reaxys, Template_relevance Reaxys_biocatalysis model, leveraging a vast database of chemical reactions to predict feasible synthetic routes.
One-Step Synthesis Focus: Specifically designed for one-step synthesis, it provides concise and direct routes for your target compounds, streamlining the synthesis process.
Accurate Predictions: Utilizing the extensive PISTACHIO, BKMS_METABOLIC, PISTACHIO_RINGBREAKER, REAXYS, REAXYS_BIOCATALYSIS database, our tool offers high-accuracy predictions, reflecting the latest in chemical research and data.
Strategy Settings
Precursor scoring | Relevance Heuristic |
---|---|
Min. plausibility | 0.01 |
Model | Template_relevance |
Template Set | Pistachio/Bkms_metabolic/Pistachio_ringbreaker/Reaxys/Reaxys_biocatalysis |
Top-N result to add to graph | 6 |
Feasible Synthetic Routes
Q1: What is the molecular formula and weight of 3,5,6-Trichloro-2-pyridinol?
A1: 3,5,6-Trichloro-2-pyridinol has a molecular formula of C5H2Cl3NO and a molecular weight of 200.44 g/mol.
Q2: How is 3,5,6-Trichloro-2-pyridinol typically quantified in biological and environmental samples?
A2: Several analytical methods have been developed for 3,5,6-Trichloro-2-pyridinol quantification, with gas chromatography coupled with electron capture detection (GC-ECD) and gas chromatography-mass spectrometry (GC-MS) being commonly employed. [, ] These methods typically involve extraction, derivatization, and separation steps. For instance, in one study, researchers extracted 3,5,6-Trichloro-2-pyridinol from elm bark, litter, and soil using methanol under acidic conditions, followed by liquid-liquid partitioning cleanup, derivatization with diazomethane, and analysis using GC-ECD. [] Another study employed a semiautomated method for quantifying 3,5,6-Trichloro-2-pyridinol in human urine using a laboratory robotics system for sample preparation, followed by extraction, derivatization, and analysis by GC-MS. []
Q3: What are some recent advancements in analytical techniques for 3,5,6-Trichloro-2-pyridinol determination?
A3: More recently, ultra-performance liquid chromatography coupled with tandem mass spectrometry (UPLC-MS/MS) has emerged as a sensitive and specific method for quantifying 3,5,6-Trichloro-2-pyridinol in human urine. [, ] This method offers advantages such as reduced analysis time and enhanced sensitivity compared to traditional GC-based methods. Additionally, enzyme-linked immunosorbent assays (ELISAs) have been developed for rapid and sensitive detection of 3,5,6-Trichloro-2-pyridinol in water samples. [, ]
Q4: How persistent is 3,5,6-Trichloro-2-pyridinol in the environment?
A4: 3,5,6-Trichloro-2-pyridinol exhibits varying persistence depending on environmental factors such as soil type, pH, and microbial activity. [, ] In one study, researchers observed that the degradation rate of 3,5,6-Trichloro-2-pyridinol decreased with soil depth, with a half-life (DT50) ranging from 42 to 49 days in surface soils and 64 to 117 days in subsurface soils. [] This suggests that 3,5,6-Trichloro-2-pyridinol can persist longer in deeper soil layers, potentially posing a risk to groundwater resources.
Q5: What are the potential pathways for biodegradation of 3,5,6-Trichloro-2-pyridinol?
A5: Several bacterial and fungal strains have been identified that can degrade 3,5,6-Trichloro-2-pyridinol. [, , , , ] One study, for instance, reported the isolation of a bacterial strain, Ochrobactrum sp. CPD-03, from a paddy field, which could degrade 3,5,6-Trichloro-2-pyridinol in minimal salt medium. [] Genome analysis revealed the presence of genes encoding enzymes such as arylesterase, suggesting a potential pathway for 3,5,6-Trichloro-2-pyridinol mineralization. Another study identified a gene cluster, dhpRIJK, in Ralstonia sp. strain T6 involved in 3,5,6-Trichloro-2-pyridinol degradation. [] The enzyme DhpJ was found to transform a metabolite of 3,5,6-Trichloro-2-pyridinol, 3,6-dihydroxypyridine-2,5-dione, further down the degradation pathway. These findings highlight the potential for bioremediation of 3,5,6-Trichloro-2-pyridinol-contaminated environments.
Q6: What are the potential toxic effects of 3,5,6-Trichloro-2-pyridinol?
A6: While generally considered less toxic than its parent compound chlorpyrifos, 3,5,6-Trichloro-2-pyridinol has been shown to induce oxidative stress and DNA damage in earthworms (Eisenia fetida). [] In a study evaluating the toxicity of 3,5,6-Trichloro-2-pyridinol in different soils, researchers observed that the compound induced excessive reactive oxygen species and caused DNA damage in earthworms, similar to chlorpyrifos. [] This suggests that 3,5,6-Trichloro-2-pyridinol may pose ecological risks, particularly in soil ecosystems.
Q7: Can 3,5,6-Trichloro-2-pyridinol be used as a biomarker of chlorpyrifos exposure?
A7: Yes, 3,5,6-Trichloro-2-pyridinol is considered a specific and sensitive biomarker for assessing exposure to chlorpyrifos and chlorpyrifos-methyl. [, , , , , ] Numerous studies have employed the measurement of 3,5,6-Trichloro-2-pyridinol levels in urine as an indicator of chlorpyrifos exposure in both occupational and environmental settings. [, , , , ] For example, a study investigating pesticide exposure among farmworkers in North Carolina found a high prevalence of detectable 3,5,6-Trichloro-2-pyridinol levels in urine samples, highlighting the compound's utility as a biomarker for assessing pesticide exposure in agricultural workers. []
試験管内研究製品の免責事項と情報
BenchChemで提示されるすべての記事および製品情報は、情報提供を目的としています。BenchChemで購入可能な製品は、生体外研究のために特別に設計されています。生体外研究は、ラテン語の "in glass" に由来し、生物体の外で行われる実験を指します。これらの製品は医薬品または薬として分類されておらず、FDAから任何の医療状態、病気、または疾患の予防、治療、または治癒のために承認されていません。これらの製品を人間または動物に体内に導入する形態は、法律により厳格に禁止されています。これらのガイドラインに従うことは、研究と実験において法的および倫理的な基準の遵守を確実にするために重要です。