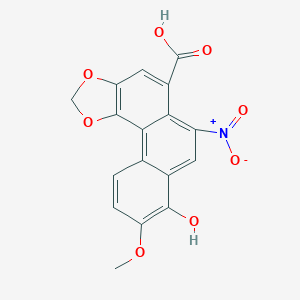
アリストロキア酸 E
概要
説明
Aristolochic acid E is a member of the aristolochic acids family, which are naturally occurring compounds found in plants of the Aristolochiaceae family, particularly in the genera Aristolochia and Asarum . These compounds are known for their potent carcinogenic and nephrotoxic properties . Aristolochic acid E, like its analogues, has been studied for its toxicological effects and its role in traditional medicine.
科学的研究の応用
Toxicological Research
Nephrotoxicity and Cancer Correlation
AAE is primarily known for its role in aristolochic acid nephropathy (AAN), a condition characterized by chronic kidney disease and a high incidence of upper urinary tract urothelial carcinoma (UUC). Studies have shown that exposure to AAE can lead to severe renal damage and mutations associated with cancer development. For instance, a significant number of patients who consumed herbal products containing AAE developed UUC, highlighting the compound's carcinogenic potential .
Mechanistic Studies
Research indicates that AAE induces DNA damage through the formation of DNA adducts, which are critical in the mutagenesis process leading to cancer. In vitro studies demonstrated that AAE is mutagenic in various cell lines, causing mutations predominantly characterized by A:T to T:A transversions . Furthermore, animal studies have confirmed that exposure to AAE results in tumors across multiple organ systems, including the kidneys and urinary tract .
Pharmacological Applications
Potential Antitumor Activity
Despite its toxicity, there is ongoing research into the potential antitumor properties of aristolochic acids, including AAE. Some studies suggest that structural modifications or combinations with other therapeutic agents might mitigate toxicity while enhancing anticancer efficacy . The exploration of AAE's immune-modulating effects is also a promising area of research, as it may provide insights into its dual role as both a toxin and a potential therapeutic agent .
Analytical Chemistry and Detection Methods
Detection Techniques
The need for accurate detection methods for AAE in herbal products has led to advancements in analytical chemistry. Improved techniques for identifying aristolochic acids in plant samples are essential for ensuring consumer safety and regulatory compliance. Research emphasizes the importance of developing rapid detection technologies to identify AA-DNA adducts in biological samples .
Case Studies and Epidemiological Evidence
Epidemiological Studies
Several case studies have documented the effects of AAE on human health. For instance, a significant number of cases were reported among patients in Belgium who consumed slimming pills containing herbal extracts with AAE . These studies highlighted the urgent need for awareness regarding the consumption of traditional medicines containing aristolochic acids.
Study | Population | Findings |
---|---|---|
Nortier et al. (2000) | Belgian patients with nephropathy | High rates of UUC (40-46%) among patients exposed to AAE |
Vanherweghem et al. (1993) | Female patients using herbal slimming pills | Rapidly progressive interstitial nephritis linked to AAE |
Liu et al. (2004) | Hupki mouse fibroblast cultures | Predominant mutations found in p53 DNA-binding domain due to AAE exposure |
作用機序
Target of Action
Aristolochic acids (AAs), including Aristolochic acid E, are a group of toxins commonly present in the plants of genus Aristolochia and Asarum . The primary targets of Aristolochic acid E are the kidneys , specifically the proximal tubule . This nephron segment is particularly prone to toxic injury due to its important role in urinary elimination of drugs and other xenobiotics .
Mode of Action
Aristolochic acid E interacts with its targets, causing necrotic and apoptotic cell death exclusively in the proximal tubule . The carcinogenic effects of aristolochic acids are thought to be a result of mutation of the tumor suppressor gene TP53 , which seems to be unique to aristolochic acid-associated carcinogenesis .
Biochemical Pathways
Aristolochic acid E affects multiple biochemical pathways. In hepatocytes, it activates NF-κB and STAT3 signaling pathways , which may contribute to the inflammatory response and apoptosis . In liver sinusoidal endothelial cells (LSECs), it activates multiple oxidative stress and inflammatory associated signaling pathways and induces apoptosis .
Pharmacokinetics
The pharmacokinetics of Aristolochic acid E involves rapid absorption and elimination, fitting into a two-compartmental open model . The elimination of Aristolochic acid E has a relationship with the dosage, with the low dose group eliminating more quickly than the high dose group .
Result of Action
The result of Aristolochic acid E’s action is severe nephrotoxicity and carcinogenicity. It can cause end-stage renal failure associated with urothelial carcinoma . The progressive lesions and mutational events initiated by Aristolochic acid E are irreversible .
Action Environment
The action of Aristolochic acid E can be influenced by environmental factors. Chronic environmental exposure to aristolochic acid due to environmental/dietary contamination has been reported . Moreover, the use of AA-containing herbal remedies and the consumption of food contaminated by AAs still carry high risk .
生化学分析
Biochemical Properties
Aristolochic Acid E interacts with various enzymes, proteins, and other biomolecules. It is metabolically activated to reactive intermediates that generate DNA adducts . The enzymes involved in this process include cytochrome P450 (CYP) 1A1 and CYP1A2 . These interactions contribute to the biochemical reactions that Aristolochic Acid E is involved in.
Cellular Effects
Aristolochic Acid E has significant effects on various types of cells and cellular processes. In hepatocytes, it activates NF-κB and STAT3 signaling pathways, which may contribute to the inflammatory response and apoptosis . In liver sinusoidal endothelial cells (LSECs), Aristolochic Acid E activates multiple oxidative stress and inflammatory associated signaling pathways and induces apoptosis .
Molecular Mechanism
Aristolochic Acid E exerts its effects at the molecular level through several mechanisms. It forms covalent DNA adducts by binding preferentially to the exocyclic amino acid group of purine nucleotides, creating a cyclic N-acylnitrenium ion with a delocalized positive charge . This leads to changes in gene expression and can result in cell damage and disease.
Temporal Effects in Laboratory Settings
The effects of Aristolochic Acid E change over time in laboratory settings. The progressive lesions and mutational events initiated by Aristolochic Acid E are irreversible
Dosage Effects in Animal Models
The effects of Aristolochic Acid E vary with different dosages in animal models. For example, in a pilot carcinogenicity study in rats, aristolochic acids were administered orally at dose levels of 0, 0.1, 1, and 10 mg/kg body weight
Metabolic Pathways
Aristolochic Acid E is involved in several metabolic pathways. It is enzymatically reduced to reactive intermediates that generate DNA adducts . The enzymes involved in this process include cytochrome P450 (CYP) 1A1 and CYP1A2 .
Transport and Distribution
Aristolochic Acid E is transported and distributed within cells and tissues. Uptake assays in heterologous expression systems identified murine organic anion transporters (mOat1, mOat2, and mOat3) as capable of mediating transport of AA-I
準備方法
Synthetic Routes and Reaction Conditions: The preparation of aristolochic acid E typically involves the extraction from natural sources, such as plants of the Aristolochiaceae family. The extraction process often includes the use of solvents like methanol or ethanol to isolate the compound from plant material .
Industrial Production Methods: Industrial production of aristolochic acid E is not common due to its toxic nature. when required for research purposes, it is usually obtained through advanced extraction techniques such as pressurized liquid extraction (PLE) and supercritical fluid extraction (SFE), which offer high efficiency and purity .
化学反応の分析
Types of Reactions: Aristolochic acid E undergoes several types of chemical reactions, including:
Oxidation: This reaction can lead to the formation of various oxidized derivatives.
Reduction: Reduction of aristolochic acid E can produce aristolactam E, a known metabolite.
Substitution: Substitution reactions can occur at the nitro or methoxy groups present in the molecule.
Common Reagents and Conditions:
Oxidation: Common oxidizing agents include potassium permanganate and hydrogen peroxide.
Reduction: Reducing agents such as sodium borohydride or catalytic hydrogenation are used.
Substitution: Conditions vary depending on the substituent being introduced, but often involve the use of strong acids or bases.
Major Products:
Oxidation: Various oxidized derivatives.
Reduction: Aristolactam E.
Substitution: Substituted derivatives with altered functional groups.
類似化合物との比較
- Aristolochic acid I
- Aristolochic acid II
- Aristolactam I
- Aristolactam II
Comparison: Aristolochic acid E shares structural similarities with other aristolochic acids but differs in the specific substituents on its phenanthrene ring . This structural variation influences its reactivity and toxicity profile. For instance, aristolochic acid I and II are more commonly studied due to their higher abundance and more pronounced toxic effects .
Aristolochic acid E is unique in its specific interactions with DNA and its distinct metabolic pathways, making it a valuable compound for studying the mechanisms of aristolochic acid-induced toxicity .
生物活性
Aristolochic acid E (AAE) is a member of the aristolochic acids family, known for its biological activities, particularly its nephrotoxic and carcinogenic properties. This compound, derived from various plants in the Aristolochia genus, has been linked to severe health issues, including aristolochic acid nephropathy (AAN) and urothelial carcinoma. This article explores the biological activity of AAE, focusing on its mechanisms of action, toxicity, and related case studies.
Aristolochic acids, including AAE, exert their toxic effects through several molecular mechanisms:
- DNA Adduct Formation : AAE forms covalent bonds with DNA, leading to the creation of DNA adducts. The predominant adduct identified is 7-(deoxyadenosin-N(6)-yl)aristolactam I (dA-AAI), which is associated with mutations in critical oncogenes such as H-ras and p53. These mutations predominantly manifest as A:T to T:A transversions, which are significant in carcinogenesis .
- Induction of Mutagenesis : In vitro studies have demonstrated that AAE induces mutations in various cell lines, including Salmonella typhimurium and mammalian cells. These mutations are linked to chromosomal aberrations and sister chromatid exchanges .
- Nephrotoxicity : AAE has been shown to cause acute kidney injury characterized by interstitial fibrosis and tubular atrophy. This nephrotoxicity is often attributed to mitochondrial dysfunction and oxidative stress induced by the compound .
- Hepatotoxicity : Emerging research indicates that AAE may also induce hepatotoxic effects, potentially leading to hepatocellular carcinoma. The mechanisms remain under investigation but involve similar pathways as those affecting renal tissues .
Case Studies
Several case studies highlight the severe consequences of AAE exposure:
- Aristolochic Acid Nephropathy (AAN) : Reports indicate over 100 cases of AAN linked to herbal products containing aristolochic acids. Patients typically presented with rapid renal failure following exposure, with many developing upper tract urothelial carcinoma .
- Carcinogenicity Evidence : Animal studies have shown that exposure to AAE leads to a high incidence of tumors in various organs. For instance, male Wistar rats developed urothelial carcinoma after subcutaneous injections of aristolochic acids .
Research Findings
Recent studies have employed advanced techniques such as single-cell RNA sequencing and proteomics to elucidate the effects of AAE at a cellular level:
- Cellular Response : Research has established a single-cell atlas of liver responses to AAE exposure, revealing significant alterations in gene expression associated with stress responses and apoptosis .
- Protein Targeting : Activity-based protein profiling has identified key metabolic enzymes affected by AAE, indicating that it disrupts mitochondrial function and cellular respiration pathways .
Summary of Biological Activities
The following table summarizes the biological activities associated with Aristolochic Acid E:
特性
IUPAC Name |
8-hydroxy-9-methoxy-6-nitronaphtho[2,1-g][1,3]benzodioxole-5-carboxylic acid | |
---|---|---|
Source | PubChem | |
URL | https://pubchem.ncbi.nlm.nih.gov | |
Description | Data deposited in or computed by PubChem | |
InChI |
InChI=1S/C17H11NO8/c1-24-11-3-2-7-8(15(11)19)4-10(18(22)23)13-9(17(20)21)5-12-16(14(7)13)26-6-25-12/h2-5,19H,6H2,1H3,(H,20,21) | |
Source | PubChem | |
URL | https://pubchem.ncbi.nlm.nih.gov | |
Description | Data deposited in or computed by PubChem | |
InChI Key |
ZRCMNWRTDNPVFL-UHFFFAOYSA-N | |
Source | PubChem | |
URL | https://pubchem.ncbi.nlm.nih.gov | |
Description | Data deposited in or computed by PubChem | |
Canonical SMILES |
COC1=C(C2=CC(=C3C(=CC4=C(C3=C2C=C1)OCO4)C(=O)O)[N+](=O)[O-])O | |
Source | PubChem | |
URL | https://pubchem.ncbi.nlm.nih.gov | |
Description | Data deposited in or computed by PubChem | |
Molecular Formula |
C17H11NO8 | |
Source | PubChem | |
URL | https://pubchem.ncbi.nlm.nih.gov | |
Description | Data deposited in or computed by PubChem | |
DSSTOX Substance ID |
DTXSID40147983 | |
Record name | Aristolochic acid E | |
Source | EPA DSSTox | |
URL | https://comptox.epa.gov/dashboard/DTXSID40147983 | |
Description | DSSTox provides a high quality public chemistry resource for supporting improved predictive toxicology. | |
Molecular Weight |
357.3 g/mol | |
Source | PubChem | |
URL | https://pubchem.ncbi.nlm.nih.gov | |
Description | Data deposited in or computed by PubChem | |
CAS No. |
107259-48-3 | |
Record name | Aristolochic acid E | |
Source | ChemIDplus | |
URL | https://pubchem.ncbi.nlm.nih.gov/substance/?source=chemidplus&sourceid=0107259483 | |
Description | ChemIDplus is a free, web search system that provides access to the structure and nomenclature authority files used for the identification of chemical substances cited in National Library of Medicine (NLM) databases, including the TOXNET system. | |
Record name | Aristolochic acid E | |
Source | EPA DSSTox | |
URL | https://comptox.epa.gov/dashboard/DTXSID40147983 | |
Description | DSSTox provides a high quality public chemistry resource for supporting improved predictive toxicology. | |
Retrosynthesis Analysis
AI-Powered Synthesis Planning: Our tool employs the Template_relevance Pistachio, Template_relevance Bkms_metabolic, Template_relevance Pistachio_ringbreaker, Template_relevance Reaxys, Template_relevance Reaxys_biocatalysis model, leveraging a vast database of chemical reactions to predict feasible synthetic routes.
One-Step Synthesis Focus: Specifically designed for one-step synthesis, it provides concise and direct routes for your target compounds, streamlining the synthesis process.
Accurate Predictions: Utilizing the extensive PISTACHIO, BKMS_METABOLIC, PISTACHIO_RINGBREAKER, REAXYS, REAXYS_BIOCATALYSIS database, our tool offers high-accuracy predictions, reflecting the latest in chemical research and data.
Strategy Settings
Precursor scoring | Relevance Heuristic |
---|---|
Min. plausibility | 0.01 |
Model | Template_relevance |
Template Set | Pistachio/Bkms_metabolic/Pistachio_ringbreaker/Reaxys/Reaxys_biocatalysis |
Top-N result to add to graph | 6 |
Feasible Synthetic Routes
Q1: What is the chemical structure of aristolochic acid E and where was it discovered?
A1: Aristolochic acid E is a naturally occurring phenanthrene compound characterized by the presence of a nitro group. It was first isolated from the roots of the Aristolochia contorta Bunge plant. [, ] Its structure is defined as 7-methoxy-8-hydroxy-aristolochic acid. []
Q2: Apart from aristolochic acid E, what other compounds were found in the Aristolochia contorta Bunge plant?
A2: In addition to aristolochic acid E, researchers identified five other compounds within the Aristolochia contorta Bunge plant: allantoin, aristolochic acid A, magnoflorine, β-sitosterol, and daucosterol. []
試験管内研究製品の免責事項と情報
BenchChemで提示されるすべての記事および製品情報は、情報提供を目的としています。BenchChemで購入可能な製品は、生体外研究のために特別に設計されています。生体外研究は、ラテン語の "in glass" に由来し、生物体の外で行われる実験を指します。これらの製品は医薬品または薬として分類されておらず、FDAから任何の医療状態、病気、または疾患の予防、治療、または治癒のために承認されていません。これらの製品を人間または動物に体内に導入する形態は、法律により厳格に禁止されています。これらのガイドラインに従うことは、研究と実験において法的および倫理的な基準の遵守を確実にするために重要です。