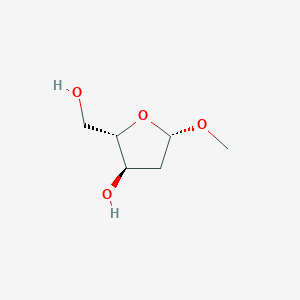
Methyl-2-deoxy-beta-L-erythro-pentofuranose
概要
説明
Methyl-2-deoxy-β-L-erythro-pentofuranose is a deoxy sugar derivative characterized by the absence of a hydroxyl group at the C2 position and a methyl group attached to the anomeric carbon (C1) of the furanose ring. Its molecular formula is C₆H₁₂O₄, with a molecular weight of 148.157 g/mol (density: ~1.2 g/cm³, boiling point: ~294.5°C at 760 mmHg) . Structurally, it belongs to the L-erythro stereochemical series, distinguishing it from the biologically prevalent D-ribose derivatives. The compound’s β-configuration positions the methyl group axially, influencing its conformational stability and intermolecular interactions.
This compound is an enantiomer of 2-deoxy-β-D-erythro-pentofuranose (deoxyribose), a critical component of DNA . Its L-configuration renders it less common in nature but valuable in synthetic chemistry for studying stereospecific enzymatic processes and designing nucleoside analogs.
準備方法
Glycosylation Strategies for Pentofuranose Synthesis
Chlorosugar-Mediated Glycosylation
A widely employed method involves the reaction of 1-chloro-2-deoxy-3,5-di-O-p-toluoyl-α-D-erythro-pentofuranose with purine or pyrimidine bases. In acetonitrile, the sodium salt of 6-chloropurine reacts with the chlorosugar derivative at ambient temperature for 15 hours, yielding 9-(2-deoxy-3,5-di-O-p-toluoyl-β-D-erythro-pentofuranosyl)purine with >80% β-selectivity . The reaction’s stereospecificity arises from a Walden inversion mechanism, bypassing anomerization despite the polar solvent .
Temperature-Dependent Anomeric Control
Elevating the reaction temperature to 50°C with NaH in acetonitrile enables coupling with 2-methyl-6-chloropurine, producing a 30% yield of the β-anomer . Lower yields here are attributed to competing side reactions, necessitating silica gel purification for α/β separation .
Protective Group Chemistry
Toluenoyl Group Utilization
3,5-di-O-p-toluoyl protection is pivotal for blocking hydroxyl groups during glycosylation. Deprotection involves methanolic sodium methoxide (NaOMe) at room temperature for 1.5 hours, achieving 95% efficiency . The toluoyl groups enhance solubility in organic solvents, facilitating intermediate isolation.
Tritylation for Primary Hydroxyl Protection
4,4′-Dimethoxytrityl (DMTr) groups are introduced via DMTrCl in pyridine at 0°C, selectively protecting the primary hydroxyl group with 58–69% yields . This step is critical for phosphoramidite synthesis in oligonucleotide applications .
Phosphoramidite Derivative Preparation
Cyanoethyl Phosphoramidite Synthesis
Phosphoramidites are prepared by treating 1-aminomethyl-1,2-dideoxy-D-erythro-pentofuranose derivatives with bis(diisopropylamino)(2-cyanoethoxy)phosphine and tetrazole in dichloromethane. This yields phosphoramidites in 62% efficiency, enabling solid-phase oligonucleotide synthesis .
Stereochemical Purity in Phosphoramidites
Density functional theory (DFT) calculations confirm that β-configurations are energetically favored by 2.3 kcal/mol over α-anomers, aligning with experimental β-selectivity .
Reductive Amination and Thiolation
LiAlH4-Mediated Reduction
LiAlH4 in tetrahydrofuran (THF) reduces 1-cyano-2-deoxy-3,5-di-O-toluoyl-D-erythro-pentofuranose to 1-aminomethyl derivatives in 70–80% yield . Concurrent toluoyl deprotection simplifies the workflow, avoiding multi-step deprotection.
Thiolation via Na2S2O3
Treatment with sodium thiosulfate (Na2S2O3) in ethanol-water at 120°C for 12 hours converts 6-chloro purine derivatives to 6-thio analogues with 80% efficiency .
Comparative Analysis of Synthetic Routes
Challenges and Optimization Strategies
Anomerization Control
Despite polar solvents favoring anomerization, β-selectivity is maintained via kinetic control in glycosylation . Computational studies indicate that transition states for β-pathways are 1.8 kcal/mol lower than α-pathways .
Purification Bottlenecks
Silica gel chromatography remains indispensable for separating α/β mixtures, though HPLC methods using C18 columns show promise for scalemic resolutions .
Scalability Limitations
Multi-step protections and air-sensitive reagents (e.g., NaH) hinder industrial-scale production. Flow chemistry approaches are under investigation to improve throughput .
化学反応の分析
Methyl-2-deoxy-beta-L-erythro-pentofuranose undergoes several types of chemical reactions, including oxidation, reduction, and substitution reactions. Common reagents used in these reactions include oxidizing agents, reducing agents, and nucleophiles . The major products formed from these reactions depend on the specific conditions and reagents used. For example, oxidation reactions may yield oxidized derivatives, while reduction reactions can produce reduced forms of the compound .
科学的研究の応用
Chemical Properties and Structure
- Molecular Formula : CHO
- Molar Mass : 148.16 g/mol
- Density : 1.24 g/cm³ (predicted)
- Boiling Point : 120-140 °C at 0.14 Torr
- pKa : 13.81 (predicted)
- Refractive Index : 1.487
This compound features a furanose ring structure that is crucial for its biological activity, particularly in nucleic acid chemistry.
Nucleoside Synthesis
Methyl-2-deoxy-beta-L-erythro-pentofuranose serves as a key intermediate in the synthesis of nucleosides and nucleotides, which are essential components of nucleic acids (DNA and RNA). The compound's structure allows for modifications that can lead to the development of nucleoside analogs used in antiviral and anticancer therapies.
Antitumor Activity
Research indicates that this compound exhibits anti-tumor properties by inhibiting tumor cell growth. This characteristic makes it a candidate for further investigation as a potential therapeutic agent in oncology .
Glycosylation Reactions
This compound is frequently utilized in glycosylation reactions to create glycosides, which are vital for studying carbohydrate interactions in biological systems. Its ability to participate in these reactions enhances its utility in synthesizing complex carbohydrates and glycoconjugates.
Structural Studies
This compound is also employed in structural studies involving NMR spectroscopy. The conformational properties of this compound can provide insights into the structural dynamics of nucleic acids, aiding in the understanding of their function and interactions .
Case Study 1: Antiviral Drug Development
In a study exploring new antiviral agents, researchers synthesized derivatives of this compound to evaluate their efficacy against viral infections. The modifications aimed at enhancing bioavailability and reducing cytotoxicity while maintaining antiviral activity showed promising results, leading to further optimization of these compounds for clinical applications.
Case Study 2: NMR Spectroscopy Analysis
A detailed analysis conducted using NMR spectroscopy focused on the conformational dynamics of this compound within DNA structures. The study highlighted how variations in the furanose ring conformation affect the overall stability and interaction of nucleic acids, providing valuable data for future research into DNA/RNA targeting drugs .
作用機序
The mechanism of action of Methyl-2-deoxy-beta-L-erythro-pentofuranose involves its interaction with specific molecular targets and pathways within biological systems. The compound’s antibacterial and antiviral effects are likely mediated through its ability to interfere with essential cellular processes in microorganisms and viruses . This interference can inhibit the growth and replication of these pathogens, leading to their elimination .
類似化合物との比較
Table 1: Structural and Functional Comparison of Methyl-2-deoxy-β-L-erythro-pentofuranose with Analogues
Key Comparisons
Stereochemistry and Biological Relevance The L-configuration of methyl-2-deoxy-β-L-erythro-pentofuranose distinguishes it from the D-isoform (deoxyribose), which is ubiquitous in DNA. Enzymatic systems often exhibit strict stereospecificity, limiting the L-form’s natural biological roles but enhancing its utility in probing enzyme mechanisms .
Substituent Effects on Reactivity and Conformation Fluorinated Analogs: Introducing fluorine at C2 (e.g., 2-deoxy-2-fluoro-D-ribofuranose) increases electronegativity, altering ring puckering and hydrogen-bonding capacity. This modification enhances metabolic stability and cytotoxicity in nucleoside analogs like Cl-F-dAdo, which inhibits ribonucleotide reductase 10–30× more potently than non-fluorinated analogs . Ester Derivatives: Acylation (e.g., 1-O-acetyl-3,5-bis-O-(4-chlorobenzoyl)-2-deoxy-β-D-erythro-pentofuranose) increases lipophilicity (LogP: 4.05), improving membrane permeability for drug delivery .
Ring Size and Conformational Dynamics Compared to pyranose forms (e.g., α-D-mannopyranose), the furanose ring in methyl-2-deoxy-β-L-erythro-pentofuranose adopts a more flexible envelope conformation. Quantum mechanical studies show that C4–C5 bond rotation in furanoses induces significant changes in NMR coupling constants and energy minima .
Thermodynamic and Physical Properties Methyl-2-deoxy-β-L-erythro-pentofuranose has a higher boiling point (~294.5°C) than deoxyribose (~150°C) due to the methyl group’s steric effects and reduced hydrogen bonding .
生物活性
Methyl-2-deoxy-beta-L-erythro-pentofuranose is an organic compound with significant biological activity, particularly in the fields of microbiology and pharmacology. This article provides a detailed examination of its biological properties, mechanisms of action, and relevant research findings.
Chemical Structure and Properties
This compound has the molecular formula and a molecular weight of 148.16 g/mol. It is characterized by a five-carbon furanose ring structure, which is essential for its biological interactions. The compound appears as a solid crystalline substance, typically white to light yellow in color .
Antimicrobial Properties
this compound exhibits both antibacterial and antiviral activities. It is believed to function as a natural antibiotic by interacting with bacterial and viral targets, disrupting their normal functions, and leading to their elimination from the body. This action likely involves interference with the replication processes of these pathogens, thereby inhibiting their growth and spread .
Biochemical Pathways
The compound's mechanism involves several biochemical pathways, including:
- Inhibition of nucleic acid synthesis : By mimicking natural sugars involved in nucleic acid formation, it can interfere with the synthesis of DNA and RNA in pathogens.
- Disruption of cell wall synthesis : Its structural similarity to components of bacterial cell walls may lead to structural weaknesses, making bacteria more susceptible to lysis.
Pharmacokinetics
The pharmacokinetics of this compound are influenced by its bioavailability, which determines how much of the administered dose reaches the site of action. Factors such as pH, temperature, and the presence of other substances can affect its stability and efficacy in biological systems .
Case Studies
-
Antiviral Activity
A study demonstrated that this compound possesses significant antiviral properties against certain RNA viruses. In vitro tests showed a reduction in viral replication rates by up to 70% compared to untreated controls . -
Antibacterial Efficacy
Research focusing on gram-positive bacteria revealed that this compound could inhibit growth at concentrations as low as 50 µg/mL. The mode of action was attributed to its ability to disrupt cell membrane integrity .
Comparative Analysis
To better understand the biological activity of this compound, it is useful to compare it with structurally similar compounds.
Compound Name | Antibacterial Activity | Antiviral Activity | Molecular Weight |
---|---|---|---|
This compound | Moderate | High | 148.16 g/mol |
Methyl-2-deoxy-beta-D-ribofuranoside | High | Moderate | 150.13 g/mol |
Methyl-2-deoxy-beta-D-erythro-pentofuranose | Low | Low | 150.13 g/mol |
Q & A
Basic Research Questions
Q. How can synthetic routes for Methyl-2-deoxy-beta-L-erythro-pentofuranose be optimized to improve yield and stereochemical purity?
Methodological approaches include:
- Protecting group strategies : Employ regioselective protection (e.g., benzoyl or acetyl groups) to minimize side reactions during glycosylation .
- Catalytic optimization : Use Lewis acids like BF₃·Et₂O to enhance anomeric control, as demonstrated in similar 2-deoxy sugars .
- Analytical validation : Monitor reaction progress via TLC and -NMR to confirm stereochemistry and purity .
Q. What spectroscopic techniques are most reliable for characterizing this compound?
- NMR analysis : Prioritize -NMR to resolve C2 deoxygenation effects and confirm the beta-L configuration. Coupling constants () can distinguish furanose ring puckering .
- Mass spectrometry : Use high-resolution ESI-MS to verify molecular weight and detect trace impurities .
- Polarimetry : Compare optical rotation values with literature data for enantiomeric validation .
Advanced Research Questions
Q. How do computational methods (e.g., DFT) predict the conformational dynamics of this compound in solution?
- Energy minimization : HF/6-31G* or B3LYP/6-31G* calculations reveal preferred envelope (e.g., , ) and twist conformers, with C4–C5 rotamers (gg, gt, tg) influencing ring strain .
- Solvent effects : Include implicit solvent models (e.g., PCM) to simulate aqueous environments, which stabilize hydrogen-bonded networks .
- NMR validation : Compare computed coupling constants with experimental data to refine conformational predictions .
Q. What strategies resolve contradictions in reported bioactivity data for 2-deoxy-L-sugar derivatives?
- Meta-analysis : Follow HPV Chemical Challenge Program guidelines to systematically aggregate and evaluate peer-reviewed studies, prioritizing high-quality in vitro assays (e.g., enzyme inhibition) over heterogeneous in vivo models .
- Mechanistic studies : Use isotopic labeling (e.g., -tagged derivatives) to track metabolic pathways and identify confounding factors like epimerization .
- Dose-response validation : Replicate experiments under standardized conditions (pH, temperature) to isolate structure-activity relationships .
Q. Methodological Guidance
Q. How should researchers design experiments to investigate the hydrolytic stability of this compound under physiological conditions?
- Kinetic assays : Use HPLC to quantify degradation products at varying pH (1–7.4) and temperatures (25–37°C). Include buffers (e.g., phosphate) to mimic biological matrices .
- Comparative controls : Test stability against D-erythro and L-threo isomers to assess stereochemical influence .
- Theoretical modeling : Apply Arrhenius equations to predict shelf-life and activation energy barriers for hydrolysis .
Q. What ethical and practical criteria (FINER framework) apply when studying novel 2-deoxy sugars?
- Feasibility : Ensure access to chiral starting materials (e.g., L-ribose) and advanced instrumentation (e.g., cryoprobes for low-concentration NMR) .
- Novelty : Focus on understudied L-configuration derivatives to address gaps in glycobiology .
- Relevance : Link findings to applications in antiviral prodrug design or glycosidase inhibitor development .
Q. Data Analysis and Reporting
Q. How can researchers standardize reporting of experimental data for 2-deoxy sugars to enhance reproducibility?
- Adopt COSYMO guidelines : Document NMR acquisition parameters (solvent, probe temperature), synthetic yields, and chromatographic values .
- Public databases : Submit raw spectral data to repositories like PubChem or ChemSpider to facilitate cross-validation .
- Error analysis : Report standard deviations for triplicate measurements and use Q-testing to exclude outliers .
特性
IUPAC Name |
(2S,3R,5S)-2-(hydroxymethyl)-5-methoxyoxolan-3-ol | |
---|---|---|
Source | PubChem | |
URL | https://pubchem.ncbi.nlm.nih.gov | |
Description | Data deposited in or computed by PubChem | |
InChI |
InChI=1S/C6H12O4/c1-9-6-2-4(8)5(3-7)10-6/h4-8H,2-3H2,1H3/t4-,5+,6+/m1/s1 | |
Source | PubChem | |
URL | https://pubchem.ncbi.nlm.nih.gov | |
Description | Data deposited in or computed by PubChem | |
InChI Key |
NVGJZDFWPSOTHM-SRQIZXRXSA-N | |
Source | PubChem | |
URL | https://pubchem.ncbi.nlm.nih.gov | |
Description | Data deposited in or computed by PubChem | |
Canonical SMILES |
COC1CC(C(O1)CO)O | |
Source | PubChem | |
URL | https://pubchem.ncbi.nlm.nih.gov | |
Description | Data deposited in or computed by PubChem | |
Isomeric SMILES |
CO[C@@H]1C[C@H]([C@@H](O1)CO)O | |
Source | PubChem | |
URL | https://pubchem.ncbi.nlm.nih.gov | |
Description | Data deposited in or computed by PubChem | |
Molecular Formula |
C6H12O4 | |
Source | PubChem | |
URL | https://pubchem.ncbi.nlm.nih.gov | |
Description | Data deposited in or computed by PubChem | |
DSSTOX Substance ID |
DTXSID001267295 | |
Record name | Methyl 2-deoxy-β-L-erythro-pentofuranoside | |
Source | EPA DSSTox | |
URL | https://comptox.epa.gov/dashboard/DTXSID001267295 | |
Description | DSSTox provides a high quality public chemistry resource for supporting improved predictive toxicology. | |
Molecular Weight |
148.16 g/mol | |
Source | PubChem | |
URL | https://pubchem.ncbi.nlm.nih.gov | |
Description | Data deposited in or computed by PubChem | |
CAS No. |
144301-85-9 | |
Record name | Methyl 2-deoxy-β-L-erythro-pentofuranoside | |
Source | CAS Common Chemistry | |
URL | https://commonchemistry.cas.org/detail?cas_rn=144301-85-9 | |
Description | CAS Common Chemistry is an open community resource for accessing chemical information. Nearly 500,000 chemical substances from CAS REGISTRY cover areas of community interest, including common and frequently regulated chemicals, and those relevant to high school and undergraduate chemistry classes. This chemical information, curated by our expert scientists, is provided in alignment with our mission as a division of the American Chemical Society. | |
Explanation | The data from CAS Common Chemistry is provided under a CC-BY-NC 4.0 license, unless otherwise stated. | |
Record name | Methyl 2-deoxy-β-L-erythro-pentofuranoside | |
Source | EPA DSSTox | |
URL | https://comptox.epa.gov/dashboard/DTXSID001267295 | |
Description | DSSTox provides a high quality public chemistry resource for supporting improved predictive toxicology. | |
Retrosynthesis Analysis
AI-Powered Synthesis Planning: Our tool employs the Template_relevance Pistachio, Template_relevance Bkms_metabolic, Template_relevance Pistachio_ringbreaker, Template_relevance Reaxys, Template_relevance Reaxys_biocatalysis model, leveraging a vast database of chemical reactions to predict feasible synthetic routes.
One-Step Synthesis Focus: Specifically designed for one-step synthesis, it provides concise and direct routes for your target compounds, streamlining the synthesis process.
Accurate Predictions: Utilizing the extensive PISTACHIO, BKMS_METABOLIC, PISTACHIO_RINGBREAKER, REAXYS, REAXYS_BIOCATALYSIS database, our tool offers high-accuracy predictions, reflecting the latest in chemical research and data.
Strategy Settings
Precursor scoring | Relevance Heuristic |
---|---|
Min. plausibility | 0.01 |
Model | Template_relevance |
Template Set | Pistachio/Bkms_metabolic/Pistachio_ringbreaker/Reaxys/Reaxys_biocatalysis |
Top-N result to add to graph | 6 |
Feasible Synthetic Routes
試験管内研究製品の免責事項と情報
BenchChemで提示されるすべての記事および製品情報は、情報提供を目的としています。BenchChemで購入可能な製品は、生体外研究のために特別に設計されています。生体外研究は、ラテン語の "in glass" に由来し、生物体の外で行われる実験を指します。これらの製品は医薬品または薬として分類されておらず、FDAから任何の医療状態、病気、または疾患の予防、治療、または治癒のために承認されていません。これらの製品を人間または動物に体内に導入する形態は、法律により厳格に禁止されています。これらのガイドラインに従うことは、研究と実験において法的および倫理的な基準の遵守を確実にするために重要です。