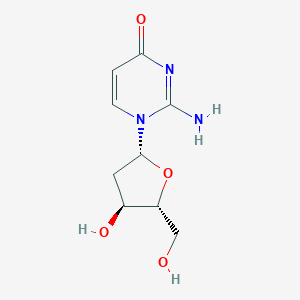
2-Amino-1-((2R,4S,5R)-4-hydroxy-5-(hydroxymethyl)tetrahydrofuran-2-yl)pyrimidin-4(1H)-one
説明
This compound, also known as 4-amino-1-(2-deoxy-β-D-erythro-pentofuranosyl)-2(1H)-pyrimidinone, is a deoxycytidine analog characterized by a pyrimidin-2-one base linked to a modified tetrahydrofuran sugar moiety. The sugar lacks a hydroxyl group at position 2, distinguishing it from natural cytidine (CAS 65-46-3) . Its molecular formula is C₉H₁₃N₃O₅, with a molecular weight of 243.22 g/mol . The absence of the 2'-OH group enhances metabolic stability compared to ribonucleosides, making it a candidate for antiviral or anticancer applications . Storage recommendations include inert atmospheres at room temperature to prevent degradation .
作用機序
Target of Action
2’-Deoxyisocytidine, also known as 2-Amino-1-((2R,4S,5R)-4-hydroxy-5-(hydroxymethyl)tetrahydrofuran-2-yl)pyrimidin-4(1H)-one, primarily targets enzymes such as Thymidine kinase 2, mitochondrial , Class B acid phosphatase , and Deoxycytidine kinase in humans . These enzymes play crucial roles in nucleotide metabolism and DNA synthesis.
Mode of Action
2’-Deoxyisocytidine is a purine nucleoside analog. It is phosphorylated into its active, triphosphate, form within the cell . The compound’s interaction with its targets leads to the inhibition of DNA synthesis and induction of apoptosis .
Biochemical Pathways
The compound affects the biochemical pathways related to DNA synthesis and cell death. By inhibiting DNA synthesis, it disrupts the normal cell cycle, leading to cell death or apoptosis . The exact biochemical pathways affected by 2’-Deoxyisocytidine are still under investigation.
Pharmacokinetics
It is known that the compound is metabolized in the body .
Result of Action
The primary result of 2’-Deoxyisocytidine’s action is the inhibition of DNA synthesis and induction of apoptosis . This leads to the death of cancer cells, particularly those in the S-phase of the cell cycle . It has broad antitumor activity targeting indolent lymphoid malignancies .
生化学分析
Biochemical Properties
2’-Deoxyisocytidine interacts with various enzymes, proteins, and other biomolecules in biochemical reactions. It is a purine nucleoside analog, and purine nucleoside analogs have broad antitumor activity targeting indolent lymphoid malignancies . The anticancer mechanisms in this process rely on inhibition of DNA synthesis and induction of apoptosis .
Cellular Effects
2’-Deoxyisocytidine has significant effects on various types of cells and cellular processes. For instance, it has been shown to induce cardiac differentiation in human umbilical cord mesenchymal stem cells . The transplanted cells differentiated into functional cardiomyocytes and significantly improved cardiac performance .
Molecular Mechanism
At the molecular level, 2’-Deoxyisocytidine exerts its effects through several mechanisms. It is incorporated into DNA, leading to premature chain termination after insertion of another nucleotide triphosphate . This non-terminal position inhibits its removal by DNA repair enzymes, ultimately leading to apoptosis .
Temporal Effects in Laboratory Settings
Over time in laboratory settings, 2’-Deoxyisocytidine exhibits changes in its effects. For instance, oligonucleotides containing 2’-Deoxyisocytidine are susceptible to a stepwise depyrimidination . Oligonucleotides incorporating 2’-O-alkylated nucleosides are stable .
Metabolic Pathways
2’-Deoxyisocytidine is involved in several metabolic pathways. It is metabolized through glycolysis and oxidative phosphorylation to produce adenosine triphosphate (ATP), which provides the energy for most cellular functions .
生物活性
2-Amino-1-((2R,4S,5R)-4-hydroxy-5-(hydroxymethyl)tetrahydrofuran-2-yl)pyrimidin-4(1H)-one, also known as 2’-deoxyisocytidine, is a nucleoside analog with significant potential in medicinal chemistry. Its unique structural features contribute to various biological activities, particularly in the context of cancer therapeutics and enzyme inhibition.
- Molecular Formula : C₉H₁₃N₃O₄
- Molecular Weight : 227.22 g/mol
- CAS Number : 123075-23-0
The biological activity of this compound primarily involves its role as a purine nucleoside analog. It is phosphorylated within cells to form its active triphosphate form, which then interferes with DNA synthesis and cellular proliferation. The compound targets several key enzymes involved in nucleotide metabolism:
- Thymidine Kinase 2 (TK2) : Involved in the phosphorylation of nucleosides, crucial for DNA synthesis.
- Deoxycytidine Kinase (dCK) : Plays a significant role in the activation of nucleoside analogs.
- Class B Acid Phosphatase : Involved in various metabolic processes that may be disrupted by the presence of the compound.
By inhibiting these enzymes, 2’-deoxyisocytidine disrupts normal cell cycle progression, leading to apoptosis in rapidly dividing cells, such as those found in tumors .
Anticancer Properties
Preliminary studies indicate that 2’-deoxyisocytidine exhibits anticancer properties by inhibiting tumor growth through several mechanisms:
- Inhibition of DNA Synthesis : Disruption of nucleotide supply leads to impaired DNA replication.
- Modulation of Signaling Pathways : The compound may alter cellular signaling pathways that regulate cell growth and survival.
Enzyme Inhibition
Research has demonstrated that this compound can inhibit specific enzymes critical for cancer cell metabolism:
Enzyme | Role | Effect of 2’-deoxyisocytidine |
---|---|---|
Thymidine Kinase 2 | Phosphorylates thymidine | Inhibition reduces nucleotide availability |
Deoxycytidine Kinase | Activates deoxynucleosides | Impairs DNA synthesis |
Class B Acid Phosphatase | Involved in metabolic regulation | Disruption may affect tumor metabolism |
Case Studies and Research Findings
Several studies have explored the biological activity and therapeutic potential of 2’-deoxyisocytidine:
-
Study on Antitumor Activity :
- In vitro studies showed that treatment with 2’-deoxyisocytidine resulted in significant apoptosis in various cancer cell lines, including breast and lung cancer models. The mechanism was linked to the inhibition of TK2 and dCK activities.
- Pharmacokinetics :
- Nucleoside Analog Development :
Q & A
Q. Basic: What are the critical steps for synthesizing this compound, and how are reaction conditions optimized?
Answer:
Synthesis typically involves multi-step organic reactions, including:
- Nucleoside coupling : Formation of the glycosidic bond between the pyrimidine base and the tetrahydrofuran moiety under controlled pH (6.5–7.5) and temperature (40–60°C) to minimize side reactions .
- Hydroxyl group protection : Use of tert-butyldimethylsilyl (TBS) or benzoyl groups to prevent undesired oxidation .
- Chromatographic purification : Reverse-phase HPLC or flash chromatography with gradients of acetonitrile/water for >95% purity .
Key Optimization Parameters :
Parameter | Range/Technique | Impact on Yield/Purity |
---|---|---|
Temperature | 40–60°C | Higher yields at 50°C |
pH | 6.5–7.5 (buffered) | Prevents hydrolysis |
Solvent System | Acetonitrile/water (HPLC) | Resolves stereoisomers |
Q. Basic: How is the molecular structure confirmed, and what analytical techniques are prioritized?
Answer:
Structural validation employs:
- NMR Spectroscopy : - and -NMR to confirm stereochemistry (e.g., 2R,4S,5R configuration) and hydrogen bonding patterns .
- X-ray Crystallography : SHELX programs for refining crystal structures, with R-factors <0.05 for high-resolution data .
- Mass Spectrometry : High-resolution MS (HRMS) to verify molecular weight (e.g., [M+H] at 263.20 m/z) .
Q. Advanced: What is the proposed mechanism of action in antiviral studies?
Answer:
The compound inhibits viral polymerases (e.g., RNA/DNA-dependent enzymes) via:
- Competitive binding : Mimics natural nucleosides, incorporating into viral nucleic acids and causing chain termination .
- Enzyme kinetics assays : IC values measured using fluorescence-based polymerase activity assays (e.g., IC = 0.5–2 µM for HCV NS5B) .
Q. Advanced: How are contradictions in bioactivity data across studies resolved?
Answer: Discrepancies arise from:
- Cell line variability : Test in multiple models (e.g., HeLa, HepG2) with standardized protocols .
- Solubility differences : Use DMSO stock solutions (<0.1% final concentration) to avoid cytotoxicity .
- Statistical rigor : Apply ANOVA with post-hoc Tukey tests (p<0.05) to validate significance .
Q. Advanced: What challenges arise in crystallographic refinement, and how are they addressed?
Answer: Common issues include:
- Disorder in the hydroxymethyl group : Resolved using SHELXL restraints (e.g., DFIX for bond lengths) .
- Twinned crystals : Implement HKLF5 format in SHELX for twin-law refinement .
- Low-resolution data : Apply TLS (Translation-Libration-Screw) models to improve R-factor convergence .
Q. Basic: What storage conditions ensure compound stability?
Answer:
- Short-term : -20°C in amber vials under argon (6 months stability) .
- Long-term : -80°C with desiccants (e.g., silica gel) to prevent hydrolysis .
- In solution : Avoid freeze-thaw cycles; prepare fresh working solutions in PBS (pH 7.4) .
Q. Advanced: How are enantiomeric impurities detected and quantified?
Answer:
- Chiral HPLC : Use columns like Chiralpak IA-3 with hexane/isopropanol (85:15) to resolve enantiomers (R >1.5) .
- Circular Dichroism (CD) : Monitor Cotton effects at 260–280 nm for stereochemical validation .
Q. Advanced: What computational methods predict target binding affinity?
Answer:
- Molecular docking : AutoDock Vina with viral polymerase PDB structures (e.g., 3KLF for HCV) .
- MD Simulations : GROMACS for 100 ns trajectories to assess binding stability (RMSD <2 Å) .
Q. Basic: How is biological activity validated in cell-based assays?
Answer:
- Cytotoxicity : MTT assay (48–72 hr exposure; IC >50 µM for selectivity) .
- Antiviral efficacy : Plaque reduction assays (e.g., 90% inhibition at 10 µM for influenza A) .
Q. Advanced: What strategies improve solubility without altering bioactivity?
Answer:
類似化合物との比較
Structural modifications in nucleoside analogs significantly influence their physicochemical properties, stability, and biological activity. Below is a comparative analysis of key analogs:
Table 1: Structural and Functional Comparison of Analogous Compounds
Key Findings from Structural Comparisons
Sugar Modifications: The 2'-deoxy group in the target compound reduces susceptibility to enzymatic degradation compared to ribonucleosides like cytidine . Dioxolane sugars (e.g., D-FDOC) mimic natural ribose conformations, improving pharmacokinetics in antiviral therapies .
Base Modifications :
- 5-Methylation (as in 5-methyl-2'-deoxycytidine) mimics epigenetic markers like 5-methylcytosine, useful in studying DNA methylation .
- Fluorination at position 5 (D-FDOC) disrupts base pairing, inhibiting viral replication .
Stability and Storage: Halogenated derivatives (e.g., CAS 95058-81-4) require storage at -20°C due to higher reactivity , whereas non-halogenated analogs (e.g., cytidine) remain stable at room temperature .
Biological Activity :
- The purine analog (CAS 123402-21-1) demonstrates broader anticancer activity due to purine's role in ATP-dependent processes .
- Chloro-fluoro derivatives (CAS 1786426-19-4) are under investigation for targeted therapies, leveraging halogen interactions with enzyme active sites .
Research Implications
- Antiviral Potential: Fluorinated analogs (e.g., D-FDOC) show promise against DNA viruses by mimicking natural nucleosides and terminating chain elongation .
- Epigenetic Studies : 5-Methyl derivatives serve as stable probes for DNA methylation mechanisms .
- Drug Design : Halogenation and sugar-ring modifications (e.g., dioxolane) optimize metabolic stability and target specificity .
特性
IUPAC Name |
2-amino-1-[(2R,4S,5R)-4-hydroxy-5-(hydroxymethyl)oxolan-2-yl]pyrimidin-4-one | |
---|---|---|
Source | PubChem | |
URL | https://pubchem.ncbi.nlm.nih.gov | |
Description | Data deposited in or computed by PubChem | |
InChI |
InChI=1S/C9H13N3O4/c10-9-11-7(15)1-2-12(9)8-3-5(14)6(4-13)16-8/h1-2,5-6,8,13-14H,3-4H2,(H2,10,11,15)/t5-,6+,8+/m0/s1 | |
Source | PubChem | |
URL | https://pubchem.ncbi.nlm.nih.gov | |
Description | Data deposited in or computed by PubChem | |
InChI Key |
HPDXILRPBZRTHG-SHYZEUOFSA-N | |
Source | PubChem | |
URL | https://pubchem.ncbi.nlm.nih.gov | |
Description | Data deposited in or computed by PubChem | |
Canonical SMILES |
C1C(C(OC1N2C=CC(=O)N=C2N)CO)O | |
Source | PubChem | |
URL | https://pubchem.ncbi.nlm.nih.gov | |
Description | Data deposited in or computed by PubChem | |
Isomeric SMILES |
C1[C@@H]([C@H](O[C@H]1N2C=CC(=O)N=C2N)CO)O | |
Source | PubChem | |
URL | https://pubchem.ncbi.nlm.nih.gov | |
Description | Data deposited in or computed by PubChem | |
Molecular Formula |
C9H13N3O4 | |
Source | PubChem | |
URL | https://pubchem.ncbi.nlm.nih.gov | |
Description | Data deposited in or computed by PubChem | |
Molecular Weight |
227.22 g/mol | |
Source | PubChem | |
URL | https://pubchem.ncbi.nlm.nih.gov | |
Description | Data deposited in or computed by PubChem | |
試験管内研究製品の免責事項と情報
BenchChemで提示されるすべての記事および製品情報は、情報提供を目的としています。BenchChemで購入可能な製品は、生体外研究のために特別に設計されています。生体外研究は、ラテン語の "in glass" に由来し、生物体の外で行われる実験を指します。これらの製品は医薬品または薬として分類されておらず、FDAから任何の医療状態、病気、または疾患の予防、治療、または治癒のために承認されていません。これらの製品を人間または動物に体内に導入する形態は、法律により厳格に禁止されています。これらのガイドラインに従うことは、研究と実験において法的および倫理的な基準の遵守を確実にするために重要です。