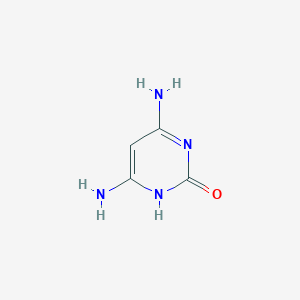
4,6-Diamino-2-hydroxypyrimidine
説明
準備方法
Synthetic Routes and Reaction Conditions: The synthesis of 4,6-Diamino-2-hydroxypyrimidine typically involves the reaction of ethyl cyanoacetate with guanidine hydrochloride in the presence of sodium ethoxide. The reaction mixture is heated under reflux, followed by acidification to yield the desired product .
Industrial Production Methods: Industrial production methods for this compound often involve similar synthetic routes but on a larger scale. The use of optimized reaction conditions and purification techniques ensures high yield and purity of the compound .
化学反応の分析
Substitution Reactions
The amino and hydroxyl groups participate in nucleophilic substitution reactions under controlled conditions:
-
Chlorination : Treatment with phosphorus oxychloride (POCl₃) replaces the hydroxyl group with chlorine, forming 2,4-diamino-6-chloropyrimidine. This reaction proceeds via electrophilic aromatic substitution, with POCl₃ acting as both a solvent and chlorinating agent .
Example : -
Hydrolysis : The chlorinated derivative can revert to the hydroxyl form under acidic or basic hydrolysis, demonstrating reversibility in substitution reactions.
Acylation Reactions
The amino groups react with acylating agents to form amide derivatives. A patented method utilizes formamide and catalytic sodium thiosulfate for efficient acylation :
Reaction Pathway :
Key Conditions :
-
Temperature gradient: 60–90°C (1–3 hours), then 95–120°C (2–4 hours) .
-
Atom economy: >85% yield with ammonium sulfate as a byproduct .
Alkylation and Condensation
The amino groups undergo alkylation and condensation to form complex heterocycles:
-
Alkylation : Reacts with alkyl halides (e.g., methyl iodide) in basic media to produce N-alkylated derivatives.
-
Schiff Base Formation : Condenses with aldehydes (e.g., formaldehyde) to form poly(azomethine-urethane) derivatives, as demonstrated in fluorescence probe synthesis .
Example :Applications : These derivatives serve as selective fluorescent probes for Cu²⁺ detection, with a limit of 7.87 × 10⁻⁶ mol/L .
Cross-Coupling Reactions
The compound’s halogenated derivatives participate in transition-metal-catalyzed reactions:
-
Suzuki Coupling : 2,4-Diamino-6-iodopyrimidine (derived from iodination) reacts with aryl boronic acids to form biaryl structures .
Conditions :
Cyclization Reactions
Under acidic conditions, intramolecular cyclization forms fused pyrimidine systems, enhancing biological activity (e.g., antitumor properties).
Mechanistic Insights
-
Hydrogen Bonding : The hydroxyl and amino groups facilitate interactions with biological targets (e.g., enzyme active sites), contributing to antifolate and antimicrobial activities.
-
Electrophilic Aromatic Substitution : Directed by electron-donating amino groups, enhancing reactivity at specific ring positions .
科学的研究の応用
Chemistry
DAHP serves as a building block in the synthesis of more complex organic molecules. Its functional groups allow for various chemical modifications, making it valuable in organic synthesis.
Biology
Research indicates that DAHP plays a potential role in DNA and RNA synthesis due to its structural similarity to nucleobases. Studies have shown that derivatives of DAHP can inhibit nitric oxide (NO) production in immune cells, suggesting possible applications in immunology and inflammation research .
Medicine
Ongoing research is exploring DAHP's potential as a therapeutic agent for treating certain cancers and viral infections. Its ability to modulate biochemical pathways makes it a candidate for drug development aimed at diseases influenced by NO levels .
Industry
In industrial applications, DAHP is utilized in the production of dyes and pigments . Its chemical properties allow it to act as an effective precursor for various industrial chemicals.
Case Study 1: Antimicrobial Activity
Research on metal complexes of DAHP has demonstrated antimicrobial properties against bacteria and fungi. These complexes were characterized through elemental analysis and various spectroscopic techniques, revealing their potential as antimicrobial agents .
Case Study 2: Inhibition of Nitric Oxide Production
A study investigated the effects of DAHP on nitric oxide production in immune-activated cells. The results indicated that DAHP significantly suppressed NO production, highlighting its potential role in anti-inflammatory therapies .
Data Table: Comparison of Applications
Application Area | Description | Key Findings |
---|---|---|
Chemistry | Building block for organic synthesis | Versatile reactions (oxidation, reduction) |
Biology | Role in DNA/RNA synthesis | Inhibits NO production in immune cells |
Medicine | Potential therapeutic agent | Promising results against cancer and viral infections |
Industry | Production of dyes/pigments | Effective precursor for industrial chemicals |
作用機序
The mechanism of action of 4,6-Diamino-2-hydroxypyrimidine involves its interaction with bacterial enzymes, leading to the inhibition of essential metabolic pathways. This results in the disruption of bacterial cell wall synthesis and ultimately bacterial cell death . The compound targets specific enzymes and pathways, making it a potent antibacterial agent .
類似化合物との比較
Chemical Identity :
- CAS No.: 31458-45-4
- Molecular Formula : C₄H₆N₄O
- Molecular Weight : 126.12 g/mol
- Structure: A pyrimidine ring with hydroxyl (-OH) at position 2 and amino (-NH₂) groups at positions 4 and 6 .
Key Properties :
- Storage : Requires storage at 2–8°C in a sealed, dry environment .
- Hazard Profile : Classified under GHS hazard statement H314 (causes severe skin burns and eye damage) .
Comparison with Structurally Similar Pyrimidine Derivatives
Substituent Variations and Reactivity
Hydroxyl vs. Thiol/Mercapto Groups
- 4,6-Diamino-2-mercaptopyrimidine (CAS 1005-59-0): Formula: C₄H₆N₄S Molecular Weight: 142.18 g/mol Key Difference: Replacement of hydroxyl (-OH) with a thiol (-SH) group. Impact: Thiol groups enhance nucleophilicity, making this compound more reactive in sulfonation and alkylation reactions compared to the hydroxyl analog .
- 4,6-Diamino-2-methylmercaptopyrimidine (Synonyms: 2-(methylthio)pyrimidine-4,6-diamine): Key Difference: Methylthio (-SCH₃) substituent at position 2. Reactivity differs due to sulfur's electron-donating effects .
Amino vs. Methoxy Groups
- 2-Amino-4,6-dimethoxypyrimidine (CAS 36315-01-2): Formula: C₆H₉N₃O₂ Molecular Weight: 155.15 g/mol Key Difference: Methoxy (-OCH₃) groups at positions 4 and 6 instead of amino (-NH₂) groups. Impact: Methoxy groups reduce polarity, altering solubility in organic solvents. This derivative is less reactive in acid-catalyzed reactions compared to amino-substituted analogs .
Positional Isomerism
- 2,6-Diamino-4-hydroxypyrimidine and 4-Amino-2,6-dihydroxypyrimidine: Structural Difference: Positions of amino and hydroxyl groups vary. Impact: Positional isomerism affects hydrogen-bonding patterns and electronic distribution. For example, 2,6-diamino-4-hydroxypyrimidine may exhibit stronger intermolecular interactions in crystal lattices due to symmetrical substitution .
Methyl-Substituted Derivatives
- 4,6-Dihydroxy-2-methylpyrimidine (CAS 40497-30-1):
- Formula : C₅H₆N₂O₂
- Molecular Weight : 126.12 g/mol
- Key Difference : Methyl (-CH₃) group at position 2 and hydroxyl groups at positions 4 and 4.
- Properties :
- Melting Point: >360°C (significantly higher than 4,6-diamino-2-hydroxypyrimidine).
Solubility : Soluble in sodium hydroxide, indicating deprotonation under basic conditions.
- 4,6-Diamino-5-methylpyrimidin-2(1H)-one (CAS 13265-41-3): Key Difference: Methyl group at position 5. Impact: Steric hindrance at position 5 may reduce accessibility for electrophilic substitution reactions .
生物活性
4,6-Diamino-2-hydroxypyrimidine (DAHP), a pyrimidine derivative, has garnered attention for its biological activities, particularly as an inhibitor of GTP cyclohydrolase I (GCH1). This compound plays a significant role in the regulation of nitric oxide (NO) synthesis and has implications in various physiological and pathological processes.
DAHP functions primarily by inhibiting GCH1, the rate-limiting enzyme in the de novo synthesis of tetrahydrobiopterin (BH4), a crucial cofactor for nitric oxide synthase (NOS). By reducing BH4 levels, DAHP effectively suppresses NO production in various cell types, including fibroblasts and endothelial cells. This inhibition is particularly relevant in inflammatory responses where NO plays a pivotal role.
Key Findings:
- Inhibition of Nitric Oxide Production : DAHP has been shown to significantly reduce NO synthesis in cytokine-activated cells, indicating its potential utility in managing conditions characterized by excessive NO production, such as inflammation and cancer .
- Dynamic Regulation of BH4 : The compound causes a rapid decrease in BH4 levels across tissues, with a half-life of less than 12 hours. Notably, BH4 levels return to baseline within 24 hours after DAHP administration, suggesting a dynamic biosynthetic regulation .
In Vitro Studies
- Fibroblast and Endothelial Cell Studies : DAHP inhibited the expression of inducible NOS (iNOS) protein and mRNA in a BH4-independent manner. This suggests that DAHP's effects extend beyond mere cofactor antagonism .
- Macrophage Activity : In chicken macrophages, DAHP suppressed NO production, further supporting its role as an anti-inflammatory agent .
In Vivo Studies
- Animal Models : Studies involving rats demonstrated that DAHP pre-treatment mitigated intestinal damage induced by non-steroidal anti-inflammatory drugs (NSAIDs) like indomethacin. This was evidenced by reduced levels of nitrosyl-haem formation, indicating decreased oxidative stress in the intestinal mucosa .
- Cancer Research : Recent studies have indicated that GCH1 inhibition by DAHP sensitizes ovarian and breast cancer cells to PARP inhibitors, suggesting potential therapeutic applications in oncology .
Research Findings Summary
Potential Applications
Given its biological activities, DAHP holds promise for various therapeutic applications:
- Anti-inflammatory Treatments : By inhibiting NO synthesis, DAHP could be beneficial in treating inflammatory diseases.
- Cancer Therapy : Its ability to sensitize certain cancer types to existing therapies may enhance treatment efficacy.
Q & A
Basic Research Questions
Q. What are the common synthetic routes for 4,6-Diamino-2-hydroxypyrimidine, and how can reaction conditions be optimized for yield?
Methodological Answer:
Synthesis typically involves condensation reactions between urea derivatives and β-diketones or β-keto esters under acidic or basic conditions. For example, 4,6-dihydroxypyrimidine derivatives are synthesized via cyclization of malonic acid derivatives with urea . Optimization strategies include:
- Catalyst selection: Use of HCl or NaOH to modulate reaction rates.
- Temperature control: Reactions often require reflux (100–120°C) to achieve high yields.
- Solvent systems: Polar aprotic solvents (e.g., DMF) improve solubility of intermediates.
- Purification: Recrystallization from hot water or ethanol is recommended for isolating pure crystals .
Safety Note: Handle with PPE (gloves, goggles) due to potential skin/eye irritation .
Q. Which spectroscopic techniques are most effective for characterizing this compound, and how are data interpreted?
Methodological Answer:
Key techniques include:
- NMR spectroscopy:
- Mass spectrometry (MS): Molecular ion peaks ([M+H]+) confirm the molecular weight (e.g., m/z 126.11 for 4,6-dihydroxy-2-methylpyrimidine ).
- IR spectroscopy: Stretching vibrations for -NH2 (~3300 cm⁻¹), -OH (~3200 cm⁻¹), and C=O (~1650 cm⁻¹) validate functional groups .
Q. How should researchers assess the stability of this compound under varying pH and temperature conditions?
Methodological Answer:
- pH stability: Perform kinetic studies in buffered solutions (pH 2–12) using HPLC or UV-Vis spectroscopy to monitor degradation over time. Pyrimidine derivatives are often stable in neutral to slightly acidic conditions but hydrolyze in strong bases .
- Thermal stability: Use thermogravimetric analysis (TGA) to determine decomposition temperatures (>300°C for methyl-substituted analogs ).
Advanced Research Questions
Q. How can researchers resolve contradictions in reported spectral data (e.g., NMR shifts) for this compound derivatives?
Methodological Answer:
- Comparative analysis: Cross-reference experimental data with computational predictions (e.g., DFT calculations for NMR chemical shifts) .
- Controlled replication: Repeat synthesis and characterization under standardized conditions (solvent, temperature, concentration) to isolate variables .
- Isotopic labeling: Use deuterated solvents to confirm assignments of exchangeable protons (e.g., -NH2, -OH) .
Q. What strategies are recommended for elucidating the mechanism of action of this compound in enzyme inhibition studies?
Methodological Answer:
- Molecular docking: Use software like AutoDock Vina to predict binding interactions with target enzymes (e.g., kinases or dihydrofolate reductase) .
- Kinetic assays: Perform Michaelis-Menten studies to determine inhibition type (competitive/non-competitive) and Ki values.
- Mutagenesis studies: Engineer enzyme active-site mutations to validate binding hypotheses .
Q. How can computational modeling optimize the biological activity of this compound derivatives?
Methodological Answer:
- QSAR modeling: Correlate structural descriptors (e.g., logP, H-bond donors) with activity data to design analogs with enhanced potency .
- ADMET prediction: Use tools like SwissADME to assess pharmacokinetic properties (e.g., solubility, CYP450 interactions) early in development .
Q. Data Contradiction Analysis
Example: Discrepancies in reported melting points (>300°C vs. 360°C) for methyl-substituted derivatives may arise from:
特性
IUPAC Name |
4,6-diamino-1H-pyrimidin-2-one | |
---|---|---|
Source | PubChem | |
URL | https://pubchem.ncbi.nlm.nih.gov | |
Description | Data deposited in or computed by PubChem | |
InChI |
InChI=1S/C4H6N4O/c5-2-1-3(6)8-4(9)7-2/h1H,(H5,5,6,7,8,9) | |
Source | PubChem | |
URL | https://pubchem.ncbi.nlm.nih.gov | |
Description | Data deposited in or computed by PubChem | |
InChI Key |
OKSVBJJXPDBPKN-UHFFFAOYSA-N | |
Source | PubChem | |
URL | https://pubchem.ncbi.nlm.nih.gov | |
Description | Data deposited in or computed by PubChem | |
Canonical SMILES |
C1=C(NC(=O)N=C1N)N | |
Source | PubChem | |
URL | https://pubchem.ncbi.nlm.nih.gov | |
Description | Data deposited in or computed by PubChem | |
Molecular Formula |
C4H6N4O | |
Source | PubChem | |
URL | https://pubchem.ncbi.nlm.nih.gov | |
Description | Data deposited in or computed by PubChem | |
DSSTOX Substance ID |
DTXSID80289371 | |
Record name | 4,6-Diamino-2-hydroxypyrimidine | |
Source | EPA DSSTox | |
URL | https://comptox.epa.gov/dashboard/DTXSID80289371 | |
Description | DSSTox provides a high quality public chemistry resource for supporting improved predictive toxicology. | |
Molecular Weight |
126.12 g/mol | |
Source | PubChem | |
URL | https://pubchem.ncbi.nlm.nih.gov | |
Description | Data deposited in or computed by PubChem | |
CAS No. |
31458-45-4 | |
Record name | 4,6-Diamino-2-pyrimidinol | |
Source | DTP/NCI | |
URL | https://dtp.cancer.gov/dtpstandard/servlet/dwindex?searchtype=NSC&outputformat=html&searchlist=60531 | |
Description | The NCI Development Therapeutics Program (DTP) provides services and resources to the academic and private-sector research communities worldwide to facilitate the discovery and development of new cancer therapeutic agents. | |
Explanation | Unless otherwise indicated, all text within NCI products is free of copyright and may be reused without our permission. Credit the National Cancer Institute as the source. | |
Record name | 4,6-Diamino-2-hydroxypyrimidine | |
Source | EPA DSSTox | |
URL | https://comptox.epa.gov/dashboard/DTXSID80289371 | |
Description | DSSTox provides a high quality public chemistry resource for supporting improved predictive toxicology. | |
Record name | 4,6-Diaminopyrimidin-2(1H)-one | |
Source | FDA Global Substance Registration System (GSRS) | |
URL | https://gsrs.ncats.nih.gov/ginas/app/beta/substances/4D5GB4JX52 | |
Description | The FDA Global Substance Registration System (GSRS) enables the efficient and accurate exchange of information on what substances are in regulated products. Instead of relying on names, which vary across regulatory domains, countries, and regions, the GSRS knowledge base makes it possible for substances to be defined by standardized, scientific descriptions. | |
Explanation | Unless otherwise noted, the contents of the FDA website (www.fda.gov), both text and graphics, are not copyrighted. They are in the public domain and may be republished, reprinted and otherwise used freely by anyone without the need to obtain permission from FDA. Credit to the U.S. Food and Drug Administration as the source is appreciated but not required. | |
試験管内研究製品の免責事項と情報
BenchChemで提示されるすべての記事および製品情報は、情報提供を目的としています。BenchChemで購入可能な製品は、生体外研究のために特別に設計されています。生体外研究は、ラテン語の "in glass" に由来し、生物体の外で行われる実験を指します。これらの製品は医薬品または薬として分類されておらず、FDAから任何の医療状態、病気、または疾患の予防、治療、または治癒のために承認されていません。これらの製品を人間または動物に体内に導入する形態は、法律により厳格に禁止されています。これらのガイドラインに従うことは、研究と実験において法的および倫理的な基準の遵守を確実にするために重要です。