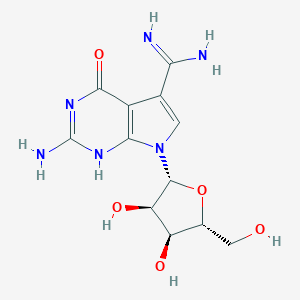
Archaeosine
概要
説明
Archaeosine (G⁺) is a hypermodified 7-deazaguanosine derivative found exclusively in archaeal tRNA, predominantly at position 15 of the D-loop . Its structure, 7-formamidino-7-deazaguanosine (Figure 1), is biosynthesized via a two-step pathway: (i) tRNA-guanine transglycosylase (ArcTGT) replaces the guanine base at position 15 with 7-cyano-7-deazaguanine (preQ₀), and (ii) this compound synthase (ArcS) converts preQ₀ to G⁺ through amidino transfer . This modification stabilizes the tRNA’s L-shaped tertiary structure by reinforcing the G15-C48 base pair, critical for thermostability in extremophiles .
準備方法
Enzymatic Synthesis of Archaeosine in Archaeal tRNA
The Three-Step Pathway: ArcTGT, ArcS, and RaSEA
This compound biosynthesis occurs via three enzymatic steps:
-
ArcTGT-mediated base exchange : The archaeal tRNA-guanine transglycosylase (ArcTGT) replaces guanine at position 15 (G15) with 7-cyano-7-deazaguanine (preQ₀) in tRNA. This reaction involves cleaving the N-glycosidic bond of G15 and forming a new bond with preQ₀ .
-
ArcS-catalyzed lysine transfer : this compound synthase (ArcS) attaches L-lysine to preQ₀, forming the intermediate preQ₀-Lys. Notably, ArcS exhibits unprecedented nucleoside-modifying activity, functioning on free nucleosides in addition to tRNA-bound substrates .
-
RaSEA-mediated cyclization : The radical S-adenosylmethionine enzyme RaSEA converts preQ₀-Lys into this compound through a radical-based mechanism, finalizing the formation of the 7-aminomethyl-7-deazaguanine structure .
Key Data :
Multisite Specificity of ArcTGT in Thermoplasma acidophilum
While most archaeal ArcTGT enzymes modify only position G15, T. acidophilum ArcTGT uniquely targets both G13 and G15 in tRNA<sup>Leu</sup>. This dual specificity was confirmed through:
-
Radiolabeling assays : Incubation of T. acidophilum S-30 cell extract with [¹⁴C]-guanine showed incorporation at positions 13 and 15 .
-
Mass spectrometry : Analysis of tRNA<sup>Leu</sup> variants expressed in Thermococcus kodakarensis revealed this compound at both sites (m/z = 455.2 for G⁺-13 and G⁺-15) .
Isolation of this compound from Native Archaeal Species
Cultivation and tRNA Extraction
This compound is typically isolated from thermophilic archaea grown under high-temperature (70–100°C) and acidic/alkaline conditions. Key protocols include:
-
Strain selection : Pyrococcus furiosus (optimal growth at 100°C) and Thermoplasma acidophilum (pH 2–3) are preferred for high this compound yields .
-
tRNA purification :
Yield Comparison :
Archaeal Species | tRNA Yield (μg/g cells) | This compound Content (pmol/μg tRNA) |
---|---|---|
P. horikoshii | 120 ± 15 | 2.8 ± 0.3 |
T. acidophilum | 95 ± 10 | 3.5 ± 0.4 |
Methanosarcina mazei | 80 ± 12 | 1.2 ± 0.2 |
In Vitro Reconstitution Using Recombinant Enzymes
Heterologous Expression of Biosynthetic Enzymes
-
ArcTGT : Expressed in E. coli BL21(DE3) Rosetta 2 with a His-tag, purified via Ni-NTA chromatography (purity >95%) .
-
ArcS : Requires co-expression with chaperones (GroEL/GroES) for solubility. Active site mutants (K128A) lose lysine transfer activity .
-
RaSEA : Oxygen-sensitive purification under anaerobic chambers (O₂ < 2 ppm) .
In Vitro Modification of tRNA Transcripts
Synthetic tRNA transcripts (e.g., T. acidophilum tRNA<sup>Leu</sup>) are modified using:
-
ArcTGT reaction : 50 mM Tris-HCl (pH 7.6), 10 mM MgCl₂, 1 mM preQ₀, 2 μM ArcTGT (30 min, 70°C) .
-
ArcS reaction : 5 mM ATP, 2 mM L-lysine, 1 μM ArcS (1 hr, 60°C) .
-
RaSEA reaction : 0.5 mM S-adenosylmethionine, 10 mM dithionite, anaerobic conditions (2 hr, 37°C) .
Efficiency : Full conversion of G15 to this compound requires a 3:1 molar ratio of ArcTGT:tRNA .
Challenges in Chemical Synthesis
Despite advances in enzymatic methods, chemical synthesis of this compound remains elusive due to:
-
Structural complexity : The 7-aminomethyl-7-deazaguanine core requires stereoselective formation of C-N bonds at C7.
-
Instability : The cyclopentenediamine moiety decomposes under acidic conditions (pH < 5) .
-
Limited demand : Most studies rely on enzymatic incorporation into tRNA rather than free nucleoside synthesis .
Heterologous Expression Systems for this compound Production
Thermococcus kodakarensis as a Model Organism
-
ΔarcTGT mutants : Show temperature-sensitive growth (no growth at 85°C vs. 95°C for wild-type) and tRNA destabilization (Tₘ reduced by 12°C) .
-
Complementation assays : Expression of T. acidophilum arcTGT restores this compound at G13/G15 and thermophily .
Table: tRNA Stability in ΔarcTGT Mutants
Condition | Tₘ (°C) Wild-Type | Tₘ (°C) ΔarcTGT | Magnesium (mM) |
---|---|---|---|
Fully modified tRNA | 82.5 ± 0.4 | 70.1 ± 0.6 | 10 |
Unmodified tRNA | 65.3 ± 0.5 | 58.9 ± 0.7 | 2 |
化学反応の分析
Types of Reactions
Archaeosine undergoes several types of chemical reactions, including:
Substitution Reactions: The initial step in this compound biosynthesis involves the substitution of guanine with preQ0.
Amidation Reactions: The conversion of preQ0 to this compound involves the amidation of a nitrile group to form a formamidine group.
Common Reagents and Conditions
Major Products
The major product of these reactions is this compound, which is incorporated into the tRNA at position 15 .
科学的研究の応用
Structural Role in tRNA Stability
Archaeosine is located at position 15 in archaeal tRNA and is essential for maintaining the three-dimensional structure of tRNA. Studies have shown that this compound contributes significantly to the stability of tRNA, particularly in thermophilic organisms like Thermococcus kodakarensis. The absence of this compound leads to a temperature-sensitive phenotype, indicating its vital role in thermal stability and proper folding of tRNA molecules .
Table 1: Impact of this compound on tRNA Stability
Organism | Effect of this compound Absence | Observed Phenotype |
---|---|---|
Thermococcus kodakarensis | Strong temperature-sensitive phenotype | Reduced growth at high temperatures |
Methanosarcina mazei | No detectable phenotype | Normal growth across temperatures |
Synthesis Pathway Insights
The synthesis of this compound involves multiple enzymatic steps. The initial step introduces a preQ0 base into tRNA via the enzyme ArcTGT. Subsequently, ArcS transfers lysine to preQ0, forming preQ0-Lys as an intermediate. Finally, RaSEA catalyzes the conversion of preQ0-Lys to this compound . Understanding this biosynthetic pathway is crucial for exploring genetic modifications and synthetic biology applications.
Table 2: Key Enzymes in this compound Synthesis
Enzyme | Function | Organism |
---|---|---|
ArcTGT | Introduces preQ0 base into tRNA | Various Archaea |
ArcS | Transfers lysine to preQ0 | Euryarchaeota |
RaSEA | Converts preQ0-Lys to this compound | Various Archaea |
Biotechnological Applications
The unique properties of this compound make it a candidate for various biotechnological applications:
- Synthetic Biology : By manipulating the this compound biosynthesis pathway, researchers can engineer organisms with enhanced thermal stability for industrial processes.
- Biomarker Development : this compound's presence can serve as a biomarker for identifying archaeal species in environmental samples, aiding in microbial ecology studies.
- Gene Therapy : Modified nucleotides like this compound may enhance the stability and efficacy of RNA-based therapeutics.
Case Study 1: Thermophilic Enzyme Activity
Research on Thermococcus kodakarensis demonstrated that deletion of genes involved in this compound synthesis resulted in significant growth deficiencies at elevated temperatures. This study highlighted the importance of this compound in maintaining enzyme activity under extreme conditions .
Case Study 2: Comparative Genomics
A comparative genomics study revealed that while many Crenarchaeota lack homologs of ArcS, they still possess G+ modifications through alternative pathways. This finding underscores the evolutionary diversity in archaeal tRNA modifications and their implications for understanding evolutionary relationships among archaea .
作用機序
Archaeosine stabilizes the tRNA structure through several distinct mechanisms. Its formamidine group, which carries a formal positive charge, interacts with the backbone phosphates of the tRNA, enhancing the stability of the tRNA tertiary structure . This stabilization is particularly important in thermophilic archaea, where high temperatures can destabilize nucleic acid structures .
類似化合物との比較
Structural and Functional Similarities to Queuosine (Q)
Queuosine (Q) is a 7-deazaguanosine derivative found in bacteria and eukaryotes at position 34 (anticodon wobble position) of tRNAs with GUN anticodons. Unlike archaeosine, Q contains a cyclopentenediol moiety (Figure 1) .
Feature | This compound (G⁺) | Queuosine (Q) |
---|---|---|
Core Structure | 7-deazaguanine + formamidino group | 7-deazaguanine + cyclopentenediol |
Location in tRNA | Position 15 (D-loop) | Position 34 (anticodon loop) |
Biosynthetic Pathway | PreQ₀ → G⁺ via ArcTGT/ArcS | PreQ₁ → Q via QueTGT/QueA/QueG |
Function | Stabilizes tRNA tertiary structure | Prevents frameshift errors |
Phylogenetic Distribution | Archaea-specific | Bacteria and eukaryotes |
Key Differences :
- Biosynthetic Substrates : this compound uses preQ₀, while Q derivatives (e.g., epoxyqueuosine) originate from preQ₁ .
- Enzymatic Machinery: ArcTGT recognizes the D-arm and aminoacyl stem of tRNA, whereas bacterial QueTGT targets the anticodon loop .
- Thermal Adaptation : this compound’s role in tRNA stabilization is critical for archaeal thermophiles, while Q optimizes translational fidelity in mesophiles .
Multisite Specificity in this compound Modifications
Most archaeal tRNAs harbor G⁺ exclusively at position 13. However, Thermoplasma acidophilum tRNALeu uniquely contains G⁺ at positions 13 and 14. This multisite specificity is attributed to its ArcTGT (Ta1493), which lacks the Zn-binding CCCH motif found in Pyrococcus horikoshii ArcTGT but retains activity through alternative substrate recognition mechanisms . Experiments with T. acidophilum cell extracts demonstrated simultaneous guanine replacement at G13 and G15, a feature absent in other archaeal ArcTGTs (e.g., P. horikoshii and Thermococcus kodakarensis ArcTGTs modify only G15) .
Comparison with Other 7-Deazaguanine Derivatives
PreQ₀ and PreQ₁ :
- PreQ₀ (7-cyano-7-deazaguanine) is the immediate precursor to this compound. Its insertion into tRNA by ArcTGT is ATP-independent .
- PreQ₁ (7-aminomethyl-7-deazaguanine) is the precursor to Q. Its incorporation by QueTGT requires ATP-dependent enzymes (QueA/QueG) for downstream modifications .
Shared Biosynthetic Steps :
Both pathways initiate with GTP cyclohydrolase I (GCH I) converting GTP to 7,8-dihydroneopterin triphosphate, a common intermediate . However, divergence occurs at preQ₀ (this compound) versus preQ₁ (Q) biosynthesis .
Evolutionary and Functional Implications
- Evolutionary Divergence : this compound and Q pathways share ancestral 7-deazaguanine synthesis enzymes (e.g., GCH I, QueD, QueE) but diverged in tRNA targeting and functional specialization .
- Thermostability vs. Translational Fidelity : this compound’s structural role contrasts with Q’s role in codon-anticodon interactions, reflecting adaptation to extreme environments versus translational accuracy .
- Enzyme Plasticity : ArcTGT’s multisite specificity in T. acidophilum underscores evolutionary flexibility in tRNA modification systems, possibly linked to niche-specific tRNA structural demands .
Data Tables
Table 2. Structural Features of ArcTGT Variants
ArcTGT Source | Zn-Binding Motif | Modification Sites | Thermal Stability |
---|---|---|---|
Pyrococcus horikoshii | Present (CCCH) | G15 only | Hyperthermophilic |
Thermoplasma acidophilum | Absent | G13 and G15 | Acidophilic/thermophilic |
生物活性
Archaeosine (G+), a modified nucleoside found in the transfer RNA (tRNA) of archaea, plays a crucial role in stabilizing tRNA structure and function. This article explores the biological activity of this compound, focusing on its synthesis, structural implications, and physiological effects in various archaeal species.
Overview of this compound
This compound is located at position 15 of many archaeal tRNAs and is synthesized from the precursor 7-cyano-7-deazaguanine (preQ0) through a series of enzymatic reactions. The key enzymes involved in this process include this compound tRNA guanine transglycosylase (ArcTGT) and this compound synthase (ArcS). The presence of this compound is particularly significant in thermophilic archaea, where it contributes to the stability of tRNA under extreme temperatures.
Synthesis Pathway
The synthesis of this compound involves multiple steps:
- Base Exchange : ArcTGT catalyzes the exchange of guanine at position 15 with preQ0.
- Lysine Transfer : ArcS facilitates the transfer of L-lysine to preQ0, forming preQ0-Lys15.
- Final Modification : A radical S-adenosyl-L-methionine enzyme converts preQ0-Lys15 into G+15.
This pathway is essential for the proper functioning of tRNA in archaea, especially those inhabiting high-temperature environments.
Stability Enhancement
Research indicates that this compound enhances the structural stability of tRNA molecules. A study on Thermococcus kodakarensis demonstrated that the absence of this compound led to a temperature-sensitive phenotype, highlighting its role in maintaining tRNA integrity under stress conditions . The stabilization effect is attributed to:
- Coulombic Interactions : The positively charged formamidine group in this compound interacts with the negatively charged backbone phosphates, strengthening the tRNA structure.
- Levitt Base Pair Formation : this compound participates in forming critical base pairs that are vital for tRNA's tertiary structure .
Physiological Effects
The biological activity of this compound extends beyond structural support; it also influences cellular fitness and growth under varying environmental conditions. In Thermococcus kodakarensis, mutants lacking this compound exhibited reduced growth rates at elevated temperatures, suggesting that this modification is crucial for survival in thermophilic habitats .
Comparative Studies
Comparative studies between different archaeal species have revealed variations in the impact of this compound on tRNA stability:
Species | Phenotype without this compound | Observations |
---|---|---|
Thermococcus kodakarensis | Temperature-sensitive | Significant growth impairment at high temperatures |
Methanosarcina mazei | No detectable phenotype | This compound loss does not affect growth significantly |
This table illustrates that while this compound is critical for some species, others may have compensatory mechanisms that mitigate its absence.
Case Studies
- Temperature Sensitivity in Thermococcus kodakarensis :
- Functional Analysis in Methanosarcina mazei :
Q & A
Q. Basic: What is the biosynthesis pathway of archaeosine (G+) in archaeal tRNA, and what experimental methods validate this process?
This compound is synthesized in two enzymatic steps: (1) ArcTGT (this compound tRNA-guanine transglycosylase) replaces the target guanine base with 7-cyano-7-deazaguanine (preQ₀), and (2) ArcS (this compound synthase) modifies preQ₀ into G+ . Validation methods include:
- Radiolabeling assays : In vitro incorporation of ¹⁴C-guanine into tRNA transcripts using purified ArcTGT or cell extracts (e.g., T. acidophilum S-30 fraction) to track base exchange activity .
- Mass spectrometry (MALDI-MS) : Direct detection of G+ modifications in purified tRNA after desalting with ZipTip C18 columns .
- Mutagenesis : Expressing tRNA variants (e.g., G13A/G15A mutants) in archaeal models like T. kodakarensis to confirm modification sites .
Q. Basic: How does ArcTGT recognize specific guanine residues in tRNA for modification?
ArcTGT targets guanines in conserved structural motifs. For example, P. horikoshii ArcTGT recognizes the ribose-phosphate backbone of the D-arm and aminoacyl stem near G15 . Experimental approaches include:
- Structural studies : X-ray crystallography of ArcTGT-tRNA complexes to map binding interfaces .
- In vitro transcription assays : Testing activity on mutant tRNA transcripts (e.g., G→A substitutions) to identify critical nucleotides .
Q. Advanced: How can researchers resolve contradictions in identifying this compound modification sites across studies?
Discrepancies arise due to species-specific tRNA structures or methodological limitations. Strategies include:
- Comparative analysis : Cross-testing ArcTGT activity from different species (e.g., P. horikoshii vs. T. acidophilum) on homologous tRNA .
- Time-course experiments : Monitoring ¹⁴C-guanine incorporation kinetics to distinguish primary vs. secondary modification sites (e.g., G13 vs. G15 in T. acidophilum tRNALeu) .
- Orthogonal validation : Combining PAGE autoradiography with MALDI-MS to confirm modifications .
Q. Advanced: What challenges exist in heterologous expression of T. acidophilum ArcTGT, and how can they be addressed?
T. acidophilum ArcTGT (Ta1493) often forms insoluble aggregates in E. coli due to misfolding. Solutions include:
- Alternative expression systems : Using thermophilic archaea like T. kodakarensis for soluble protein production .
- Domain-swapping : Replacing disordered regions (e.g., C1/C2 insertions) with stable domains from homologs .
Q. Advanced: What structural features enable T. acidophilum ArcTGT to modify multiple sites (G13 and G15) in tRNALeu?
Key determinants include:
- Flexible catalytic domain : Accommodates tRNA conformational changes during L-shaped to β-form transitions .
- Absence of Zn-binding motifs : Unlike P. horikoshii ArcTGT, T. acidophilum lacks the CCCH Zn-binding motif, potentially allowing broader substrate recognition .
- tRNA tertiary interactions : Mutagenesis shows G+13/G+15 formation depends on tRNALeu’s unique D-loop structure .
Q. Advanced: How can researchers study the functional impact of this compound modifications on tRNA stability and translation?
Methods include:
- Thermal denaturation assays : Comparing melting temperatures of wild-type vs. G+-deficient tRNA .
- Ribosome profiling : Assessing translation efficiency in archaeal strains with arcTGT knockouts .
- Molecular dynamics simulations : Modeling tRNA-ribosome interactions with/without G+ modifications .
Q. Methodological: What protocols are recommended for synthesizing this compound analogs for biochemical studies?
The Turbo-Grignard method enables efficient synthesis of deazaguanosine derivatives (e.g., preQ₀, preQ₁):
- Selective functionalization : Turbo-Grignard reagents react at specific sites without disturbing adjacent groups (e.g., amino or hydroxyl) .
- Scalability : Produces gram-scale yields for in vitro assays or structural studies .
Q. Methodological: How should researchers design experiments to analyze this compound modification kinetics?
- S-30 fraction assays : Incubate tRNA with cell extracts and ¹⁴C-guanine, then quench reactions at intervals (0–20 min) for PAGE/autoradiography .
- Quantitative LC-MS : Measure preQ₀/G+ levels over time using isotope-labeled internal standards .
Tables for Quick Reference
Key Enzymes in this compound Biosynthesis |
---|
ArcTGT |
ArcS |
Common Experimental Models |
---|
T. acidophilum |
T. kodakarensis |
特性
IUPAC Name |
2-amino-7-[(2R,3R,4S,5R)-3,4-dihydroxy-5-(hydroxymethyl)oxolan-2-yl]-4-oxo-3H-pyrrolo[2,3-d]pyrimidine-5-carboximidamide | |
---|---|---|
Source | PubChem | |
URL | https://pubchem.ncbi.nlm.nih.gov | |
Description | Data deposited in or computed by PubChem | |
InChI |
InChI=1S/C12H16N6O5/c13-8(14)3-1-18(9-5(3)10(22)17-12(15)16-9)11-7(21)6(20)4(2-19)23-11/h1,4,6-7,11,19-21H,2H2,(H3,13,14)(H3,15,16,17,22)/t4-,6-,7-,11-/m1/s1 | |
Source | PubChem | |
URL | https://pubchem.ncbi.nlm.nih.gov | |
Description | Data deposited in or computed by PubChem | |
InChI Key |
PEMQXWCOMFJRLS-RPKMEZRRSA-N | |
Source | PubChem | |
URL | https://pubchem.ncbi.nlm.nih.gov | |
Description | Data deposited in or computed by PubChem | |
Canonical SMILES |
C1=C(C2=C(N1C3C(C(C(O3)CO)O)O)N=C(NC2=O)N)C(=N)N | |
Source | PubChem | |
URL | https://pubchem.ncbi.nlm.nih.gov | |
Description | Data deposited in or computed by PubChem | |
Isomeric SMILES |
C1=C(C2=C(N1[C@H]3[C@@H]([C@@H]([C@H](O3)CO)O)O)N=C(NC2=O)N)C(=N)N | |
Source | PubChem | |
URL | https://pubchem.ncbi.nlm.nih.gov | |
Description | Data deposited in or computed by PubChem | |
Molecular Formula |
C12H16N6O5 | |
Source | PubChem | |
URL | https://pubchem.ncbi.nlm.nih.gov | |
Description | Data deposited in or computed by PubChem | |
DSSTOX Substance ID |
DTXSID801318234 | |
Record name | Archaeosine | |
Source | EPA DSSTox | |
URL | https://comptox.epa.gov/dashboard/DTXSID801318234 | |
Description | DSSTox provides a high quality public chemistry resource for supporting improved predictive toxicology. | |
Molecular Weight |
324.29 g/mol | |
Source | PubChem | |
URL | https://pubchem.ncbi.nlm.nih.gov | |
Description | Data deposited in or computed by PubChem | |
CAS No. |
148608-52-0 | |
Record name | Archaeosine | |
Source | CAS Common Chemistry | |
URL | https://commonchemistry.cas.org/detail?cas_rn=148608-52-0 | |
Description | CAS Common Chemistry is an open community resource for accessing chemical information. Nearly 500,000 chemical substances from CAS REGISTRY cover areas of community interest, including common and frequently regulated chemicals, and those relevant to high school and undergraduate chemistry classes. This chemical information, curated by our expert scientists, is provided in alignment with our mission as a division of the American Chemical Society. | |
Explanation | The data from CAS Common Chemistry is provided under a CC-BY-NC 4.0 license, unless otherwise stated. | |
Record name | Archaeosine | |
Source | ChemIDplus | |
URL | https://pubchem.ncbi.nlm.nih.gov/substance/?source=chemidplus&sourceid=0148608520 | |
Description | ChemIDplus is a free, web search system that provides access to the structure and nomenclature authority files used for the identification of chemical substances cited in National Library of Medicine (NLM) databases, including the TOXNET system. | |
Record name | Archaeosine | |
Source | EPA DSSTox | |
URL | https://comptox.epa.gov/dashboard/DTXSID801318234 | |
Description | DSSTox provides a high quality public chemistry resource for supporting improved predictive toxicology. | |
Retrosynthesis Analysis
AI-Powered Synthesis Planning: Our tool employs the Template_relevance Pistachio, Template_relevance Bkms_metabolic, Template_relevance Pistachio_ringbreaker, Template_relevance Reaxys, Template_relevance Reaxys_biocatalysis model, leveraging a vast database of chemical reactions to predict feasible synthetic routes.
One-Step Synthesis Focus: Specifically designed for one-step synthesis, it provides concise and direct routes for your target compounds, streamlining the synthesis process.
Accurate Predictions: Utilizing the extensive PISTACHIO, BKMS_METABOLIC, PISTACHIO_RINGBREAKER, REAXYS, REAXYS_BIOCATALYSIS database, our tool offers high-accuracy predictions, reflecting the latest in chemical research and data.
Strategy Settings
Precursor scoring | Relevance Heuristic |
---|---|
Min. plausibility | 0.01 |
Model | Template_relevance |
Template Set | Pistachio/Bkms_metabolic/Pistachio_ringbreaker/Reaxys/Reaxys_biocatalysis |
Top-N result to add to graph | 6 |
Feasible Synthetic Routes
試験管内研究製品の免責事項と情報
BenchChemで提示されるすべての記事および製品情報は、情報提供を目的としています。BenchChemで購入可能な製品は、生体外研究のために特別に設計されています。生体外研究は、ラテン語の "in glass" に由来し、生物体の外で行われる実験を指します。これらの製品は医薬品または薬として分類されておらず、FDAから任何の医療状態、病気、または疾患の予防、治療、または治癒のために承認されていません。これらの製品を人間または動物に体内に導入する形態は、法律により厳格に禁止されています。これらのガイドラインに従うことは、研究と実験において法的および倫理的な基準の遵守を確実にするために重要です。