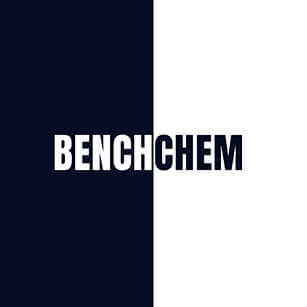
イリジウムIペンタンジオナート-シクロオクタジエン錯体
- 専門家チームからの見積もりを受け取るには、QUICK INQUIRYをクリックしてください。
- 品質商品を競争力のある価格で提供し、研究に集中できます。
説明
Iridium I Pentanedionate-Cyclo-Octadiene Complex: is a coordination compound of iridium with pentanedionate and cyclo-octadiene ligands. This compound is known for its stability and versatility, making it a valuable precursor to a variety of iridium complexes and catalysts . The molecular formula of this compound is C13H19IrO4 and it has a molecular weight of 383.51 g/mol .
科学的研究の応用
準備方法
The synthesis of Iridium I Pentanedionate-Cyclo-Octadiene Complex typically involves the reaction of a suitable iridium(I) precursor, commonly a chloro-bridged dimer such as [(cyclooctadiene)IrCl]2 , with an appropriate pentanedionate salt, often sodium pentanedionate (Na(acac)), in a suitable solvent. The reaction is usually carried out under an inert atmosphere to prevent oxidation of the iridium complex. The product is then purified by recrystallization.
化学反応の分析
Iridium I Pentanedionate-Cyclo-Octadiene Complex undergoes various types of chemical reactions, including:
Oxidation: This compound can be oxidized to form higher oxidation state iridium complexes.
Reduction: It can be reduced to form lower oxidation state iridium complexes.
Substitution: The ligands in the complex can be substituted with other ligands to form new iridium complexes.
Common reagents used in these reactions include oxidizing agents like hydrogen peroxide, reducing agents like sodium borohydride, and various ligands for substitution reactions . The major products formed from these reactions depend on the specific reagents and conditions used.
作用機序
The mechanism of action of Iridium I Pentanedionate-Cyclo-Octadiene Complex involves its ability to form stable complexes with various ligands. This stability allows it to act as a catalyst in various chemical reactions. In biological systems, iridium complexes can interact with cellular components, leading to the generation of reactive oxygen species (ROS) and induction of apoptosis in cancer cells . The molecular targets and pathways involved include DNA, proteins, and cellular signaling pathways related to oxidative stress and apoptosis .
類似化合物との比較
Iridium I Pentanedionate-Cyclo-Octadiene Complex can be compared with other similar iridium complexes, such as:
Iridium I Cyclooctadiene Tetramethylcyclopentadienyl Complex: This compound has a similar structure but with a different ligand, tetramethylcyclopentadienyl, which gives it unique properties.
Iridium I Cyclooctadiene Hexafluoroacetylacetonate Complex: This complex has hexafluoroacetylacetonate as a ligand, which affects its reactivity and stability.
The uniqueness of Iridium I Pentanedionate-Cyclo-Octadiene Complex lies in its combination of pentanedionate and cyclooctadiene ligands, which provide a balance of stability and reactivity, making it a versatile precursor for various applications.
特性
CAS番号 |
12154-84-6 |
---|---|
分子式 |
C13H19IrO 4* |
分子量 |
383.51 |
製品の起源 |
United States |
試験管内研究製品の免責事項と情報
BenchChemで提示されるすべての記事および製品情報は、情報提供を目的としています。BenchChemで購入可能な製品は、生体外研究のために特別に設計されています。生体外研究は、ラテン語の "in glass" に由来し、生物体の外で行われる実験を指します。これらの製品は医薬品または薬として分類されておらず、FDAから任何の医療状態、病気、または疾患の予防、治療、または治癒のために承認されていません。これらの製品を人間または動物に体内に導入する形態は、法律により厳格に禁止されています。これらのガイドラインに従うことは、研究と実験において法的および倫理的な基準の遵守を確実にするために重要です。