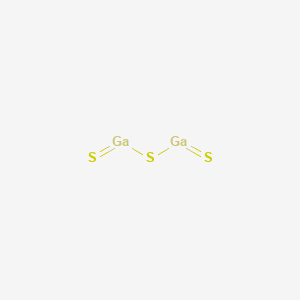
Gallium(III) sulfide
- 専門家チームからの見積もりを受け取るには、QUICK INQUIRYをクリックしてください。
- 品質商品を競争力のある価格で提供し、研究に集中できます。
説明
Gallium(III) sulfide, with the chemical formula Ga₂S₃, is a compound composed of gallium and sulfur. The compound exists in several polymorphic forms, including α (hexagonal), α’ (monoclinic), β (hexagonal), and γ (cubic), with the alpha form being yellow .
準備方法
Gallium(III) sulfide can be synthesized through various methods:
Elemental Reaction: One common method involves reacting gallium with sulfur at high temperatures.
Hydrogen Sulfide Method: Another method involves heating gallium in a stream of hydrogen sulfide gas at high temperatures.
Solid-State Reaction: This compound can also be prepared by reacting gallium chloride (GaCl₃) with sodium sulfide (Na₂S) in a solid-state reaction.
Gallium Iodide Method: A process for preparing extrapure this compound involves reacting gallium(III) iodide with sulfur in an evacuated two-zone furnace.
化学反応の分析
Gallium(III) sulfide undergoes various chemical reactions:
Oxidation and Reduction: It can be oxidized or reduced under specific conditions.
Acid Reactions: This compound dissolves in aqueous acids, slowly decomposing in moist air to form hydrogen sulfide (H₂S).
Reactions with Potassium Sulfide: It dissolves in aqueous solutions of potassium sulfide (K₂S) to form complex anions such as K₈Ga₄S₁₀.
Formation of Ternary Sulfides: This compound reacts with metal sulfides to form ternary sulfides like CdGa₂S₄.
科学的研究の応用
Gallium(III) sulfide has a wide range of scientific research applications:
Optoelectronics: It is used in the fabrication of photodetectors, field-effect transistors, and other optoelectronic devices.
Energy Storage: The compound is utilized in energy storage devices, including lithium-ion batteries.
Catalysis: This compound nanostructures have been employed as hydrogen evolution catalysts.
Thin Films: It is used in the preparation of thin films for electronic and solar cell applications.
Gas Sensors: The compound is also used in the fabrication of gas sensors on both flexible and rigid substrates.
作用機序
The mechanism of action of gallium(III) sulfide in various applications involves its unique physical and chemical properties:
Semiconductor Properties: Its wide band gap and layered structure make it suitable for use in electronic and optoelectronic devices.
Catalytic Activity: In catalysis, this compound acts as a catalyst for hydrogen evolution reactions, leveraging its surface properties and reactivity.
Energy Storage: In energy storage applications, this compound undergoes intercalation and conversion reactions with sodium ions, facilitating energy storage and release.
類似化合物との比較
Gallium(III) sulfide can be compared with other similar compounds:
Gallium(II) Sulfide (GaS): Unlike this compound, gallium(II) sulfide contains gallium in the +2 oxidation state and has different structural and electronic properties.
Zinc Sulfide (ZnS): This compound shares structural similarities with zinc sulfide, particularly in its gamma form, which is isostructural with sphalerite (zinc blende).
Aluminium Sulfide (Al₂S₃): The alpha and beta forms of this compound are isostructural with their aluminium analogues, highlighting similarities in crystal structure.
生物活性
Gallium(III) sulfide (Ga₂S₃) is a compound that has garnered attention for its unique biological activities, particularly in the fields of oncology and antimicrobial treatment. This article explores the biological properties of Ga₂S₃, including its mechanisms of action, cytotoxicity, and potential therapeutic applications.
This compound is a semiconductor material that exhibits a range of properties beneficial for various applications, including electronics and photonics. However, its biological activity, particularly as an antitumor and antimicrobial agent, is increasingly being recognized. The compound's ability to mimic iron (Fe³⁺) ions allows it to interfere with biological processes that rely on iron, making it a candidate for therapeutic use.
2.1 Iron Mimicry
Gallium(III) ions share similar properties with iron ions, such as ionic radius and electron affinity. This similarity allows Ga³⁺ to substitute for Fe³⁺ in biological systems, disrupting iron-dependent metabolic processes. For instance, Ga³⁺ can inhibit enzymes that require iron as a cofactor, leading to impaired bacterial growth and metabolism .
2.2 Antitumor Activity
Research indicates that Ga³⁺ disrupts cellular iron homeostasis, which is crucial for tumor cell proliferation. By inhibiting iron uptake and utilization within cancer cells, Ga³⁺ can induce apoptosis and reduce tumor growth. Studies have shown that gallium compounds can effectively target various cancer cell lines, including those resistant to conventional therapies .
3. Cytotoxicity Studies
Cytotoxicity assessments of this compound reveal significant effects on different cell lines. A study demonstrated that Ga₂S₃ exhibits dose-dependent cytotoxicity against human cancer cell lines, with IC₅₀ values indicating substantial cell death at relatively low concentrations .
Table 1: Cytotoxic Effects of this compound on Various Cell Lines
Cell Line | IC₅₀ (µM) | Mechanism of Action |
---|---|---|
HeLa (Cervical) | 25 | Inhibition of iron-dependent enzymes |
MCF-7 (Breast) | 30 | Induction of oxidative stress |
A549 (Lung) | 20 | Disruption of cellular metabolism |
4. Antimicrobial Properties
Gallium compounds have been shown to possess notable antimicrobial activity against a variety of pathogens. The mechanism involves the inhibition of bacterial iron acquisition systems; bacteria are unable to differentiate between Fe³⁺ and Ga³⁺, leading to disrupted metabolic functions essential for survival .
4.1 Case Study: Antibacterial Efficacy
In a study involving Pseudomonas aeruginosa, Ga³⁺ demonstrated significant bactericidal activity at micromolar concentrations. The presence of gallium led to reduced biofilm formation and altered protein expression profiles related to iron metabolism .
5. Toxicological Considerations
While gallium compounds exhibit promising therapeutic potential, they also pose risks regarding toxicity. This compound is classified as a hazardous substance with severe corrosive effects on skin and mucous membranes . Chronic exposure may lead to adverse health effects such as dermatitis and potential renal damage.
Table 2: Toxicological Profile of this compound
Exposure Route | Effects |
---|---|
Ingestion | Corrosive effects on gastrointestinal tract |
Inhalation | Respiratory irritation |
Skin Contact | Severe burns |
6. Conclusion
This compound presents significant promise in the fields of oncology and antimicrobial therapy due to its unique biological activities stemming from its ability to mimic iron ions. Ongoing research into its mechanisms of action and safety profile will be crucial in determining its viability as a therapeutic agent.
Q & A
Basic Research Questions
Q. What are the primary synthesis routes for Ga₂S₃, and how do they influence structural properties?
Ga₂S₃ can be synthesized via direct reaction of gallium with sulfur at high temperatures (e.g., 16 Ga + 3 S₈ → 8 Ga₂S₃) . Alternative methods include hydrothermal synthesis of GaOOH precursors followed by H₂S annealing and mechanochemical ball milling, which produces metastable β- and γ-polymorphs . The choice of method affects crystallinity, phase purity, and particle morphology, critical for applications in semiconductors or energy storage.
Q. How do Ga₂S₃ polymorphs differ, and what techniques are used to characterize them?
Ga₂S₃ exists in α (hexagonal), α’ (monoclinic), β (hexagonal), and γ (cubic) polymorphs, with phase transitions observed under thermal treatment . X-ray diffraction (XRD) and Raman spectroscopy are essential for distinguishing phases, while transmission electron microscopy (TEM) reveals nanostructural features like layered arrangements in β-Ga₂S₃ . Differential scanning calorimetry (DSC) can track phase transitions .
Q. What safety protocols are critical when handling Ga₂S₃ in lab settings?
Ga₂S₃ poses hazards (H314-H318: skin/eye corrosion and respiratory irritation) . Use glove boxes for synthesis, fume hoods for H₂S annealing steps , and personal protective equipment (PPE) including nitrile gloves and safety goggles. Waste disposal must comply with regulations for sulfides.
Advanced Research Questions
Q. How does Ga₂S₃ function as a sodium-ion battery (SIB) anode, and what challenges exist in optimizing its performance?
Ga₂S₃ exhibits a high theoretical capacity (~892 mAh/g) via conversion reactions (Ga₂S₃ + 6 Na⁺ → 2 Ga + 3 Na₂S) . However, volume expansion during cycling degrades stability. Strategies include nanostructuring (e.g., nanorods to buffer stress) and composite design with carbon matrices. In-situ XRD and galvanostatic intermittent titration (GITT) are used to study sodiation mechanisms and kinetics .
Q. What contradictions exist in reported bandgap values for Ga₂S₃, and how can they be resolved?
Bandgap values vary between 2.4–3.5 eV depending on phase (β-Ga₂S3: ~2.53 eV; α-phase: ~3.2 eV) and synthesis defects . Discrepancies arise from differences in characterization methods (UV-Vis vs. photoluminescence) and sample purity. Researchers should standardize synthesis protocols and employ multiple techniques (e.g., spectroscopic ellipsometry) for cross-validation .
Q. How can mechanochemical synthesis be optimized to stabilize Ga₂S₃ metastable phases for optoelectronic applications?
Mechanochemical methods produce γ-Ga₂S₃, which transitions to α’ at ~400°C . To stabilize γ-phase, use low-temperature annealing (<300°C) and explore doping (e.g., Zn or In) to inhibit phase transitions. Pair with TEM and in-situ XRD to monitor structural evolution during synthesis .
Q. What role do sulfur vacancies play in Ga₂S₃’s electronic properties, and how can they be quantified?
Sulfur vacancies act as electron donors, reducing resistivity and enhancing n-type conductivity . Positron annihilation spectroscopy and X-ray photoelectron spectroscopy (XPS) quantify vacancy density, while density functional theory (DFT) simulations model their impact on band structure .
Q. Methodological Guidance
Q. How should researchers design experiments to study Ga₂S₃’s electrochemical stability in aqueous vs. non-aqueous electrolytes?
- Non-aqueous systems : Use coin cells with NaPF₆ in ethylene carbonate/dimethyl carbonate (EC/DMC) .
- Aqueous systems : Test in neutral buffers (pH 7) to avoid Ga₂S₃ hydrolysis. Electrochemical impedance spectroscopy (EIS) and cyclic voltammetry (CV) assess interfacial stability, while inductively coupled plasma (ICP) monitors gallium dissolution .
Q. What best practices ensure reproducibility in Ga₂S₃ thin-film deposition on semiconductor substrates?
- Substrate preparation : Clean GaP or Si wafers with HF etching to remove oxides .
- Deposition : Use pulsed laser deposition (PLD) or atomic layer deposition (ALD) under controlled sulfur partial pressure .
- Characterization : Atomic force microscopy (AFM) for surface roughness; XRD for epitaxial alignment .
Q. How can DFT simulations complement experimental studies of Ga₂S₃’s sodium-ion storage mechanisms?
DFT models predict sodiation pathways, activation barriers, and volume changes . Pair with operando XRD or TEM to validate simulations. Open-source codes (e.g., VASP or Quantum ESPRESSO) are recommended for modeling .
Q. Data Interpretation and Reporting
-
Key Property Table for Ga₂S₃ :
Property Value/Range Method/Conditions Bandgap (eV) 2.4–3.5 UV-Vis, Photoluminescence Density (g/cm³) 3.65–3.77 Pycnometry Melting Point (°C) 1255 DSC Hazard Classification H314-H318 SDS compliance -
References : Cite primary literature (e.g., synthesis protocols , electrochemical studies ) and avoid over-reliance on vendor data. Use tools like Zotero for managing citations .
特性
IUPAC Name |
sulfanylidene(sulfanylidenegallanylsulfanyl)gallane |
Source
|
---|---|---|
Source | PubChem | |
URL | https://pubchem.ncbi.nlm.nih.gov | |
Description | Data deposited in or computed by PubChem | |
InChI |
InChI=1S/2Ga.3S |
Source
|
Source | PubChem | |
URL | https://pubchem.ncbi.nlm.nih.gov | |
Description | Data deposited in or computed by PubChem | |
InChI Key |
RCKBMGHMPOIFND-UHFFFAOYSA-N |
Source
|
Source | PubChem | |
URL | https://pubchem.ncbi.nlm.nih.gov | |
Description | Data deposited in or computed by PubChem | |
Canonical SMILES |
S=[Ga]S[Ga]=S |
Source
|
Source | PubChem | |
URL | https://pubchem.ncbi.nlm.nih.gov | |
Description | Data deposited in or computed by PubChem | |
Molecular Formula |
Ga2S3 |
Source
|
Record name | gallium(III) sulfide | |
Source | Wikipedia | |
URL | https://en.wikipedia.org/wiki/Gallium(III)_sulfide | |
Description | Chemical information link to Wikipedia. | |
Source | PubChem | |
URL | https://pubchem.ncbi.nlm.nih.gov | |
Description | Data deposited in or computed by PubChem | |
Molecular Weight |
235.6 g/mol |
Source
|
Source | PubChem | |
URL | https://pubchem.ncbi.nlm.nih.gov | |
Description | Data deposited in or computed by PubChem | |
試験管内研究製品の免責事項と情報
BenchChemで提示されるすべての記事および製品情報は、情報提供を目的としています。BenchChemで購入可能な製品は、生体外研究のために特別に設計されています。生体外研究は、ラテン語の "in glass" に由来し、生物体の外で行われる実験を指します。これらの製品は医薬品または薬として分類されておらず、FDAから任何の医療状態、病気、または疾患の予防、治療、または治癒のために承認されていません。これらの製品を人間または動物に体内に導入する形態は、法律により厳格に禁止されています。これらのガイドラインに従うことは、研究と実験において法的および倫理的な基準の遵守を確実にするために重要です。