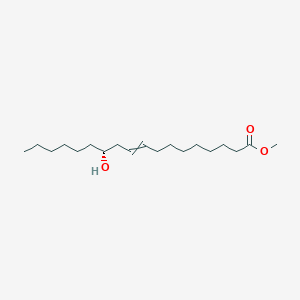
Methyl ricinoleate
概要
説明
Methyl ricinoleate is a clear, viscous fluid that is used as a surfactant, cutting fluid additive, lubricant, and plasticizer . It is a type of fatty acid methyl ester synthesized from castor oil and methyl alcohol . It is also a plasticizer for cellulosic resins, polyvinyl acetate, and polystyrene . It is derived from natural raw materials and is completely biodegradable .
Synthesis Analysis
Methyl ricinoleate is commercially obtained by the transesterification from castor oil . It has been synthesized in various studies, including one where it was produced via the environmentally friendly synthesis and photopolymerization for biomedical applications . Another study reported its production through the hydrogenation process using a Cu/Ni bimetallic catalyst .
Molecular Structure Analysis
The molecular formula of Methyl ricinoleate is C19H36O3 . Its structure has been analyzed in various studies .
Chemical Reactions Analysis
Methyl ricinoleate has been studied in various chemical reactions. For instance, the kinetics of its enzymatic hydrolysis in the presence of Candida antarctica Lipase B was analyzed . Another study investigated the product distribution arising from its pyrolysis at 550 °C .
Physical And Chemical Properties Analysis
Methyl ricinoleate has a molecular weight of 312.5 g/mol . It has a density of 0.9236 g/mL at 22 °C and a melting point of -4.5 °C . Its solubility in water is 9.87×10^-2 mg/L .
科学的研究の応用
Biomass Pyrolysis
Methyl ricinoleate (MR): is utilized in biomass pyrolysis, a process that converts organic materials into smaller molecules through thermal decomposition. MR’s pyrolysis at 550°C with varying heating rates produces valuable chemicals like undecylenic acid methyl ester (UAME) and heptanal (HEP) . UAME is used in engineering plastics, while HEP is a precursor for fragrances and flavors. The bond dissociation energies (BDEs) indicate that the C11–C12 bond in MR is the weakest, leading to these products .
Renewable Chemical Resources
The pyrolysis products of MR, such as UAME, serve as renewable resources for the chemical industry. UAME’s bifunctional nature makes it suitable for producing Nylon 11 , a type of engineering plastic . This application underscores MR’s role in creating sustainable materials.
Pharmaceutical Applications
MR is recognized in pharmaceutical applications, particularly as a secondary standard in analytical processes like quality control testing and calibration requirements . Its consistency and purity make it an ideal candidate for ensuring the accuracy of pharmaceutical analyses.
Surfactant and Lubricant
As a clear, viscous fluid, MR is used as a surfactant and lubricant . Its properties allow it to reduce surface tension in liquids, making it valuable in creating emulsions for various products. Additionally, it serves as a lubricant, providing smooth operation in mechanical systems.
Cutting Fluid Additive
MR’s application extends to being a cutting fluid additive . In machining and metalworking, cutting fluids are essential for cooling and lubricating the cutting tool and workpiece. MR enhances the performance of these fluids, improving tool life and surface finish.
Antioxidant Properties
MR exhibits antioxidant activities, which are crucial in scavenging free radicals . This property is significant in the food industry and in the development of health supplements, where antioxidants play a vital role in preserving the quality and nutritional value of products.
Safety and Hazards
Methyl ricinoleate should be handled with care. Avoid dust formation, breathing mist, gas or vapours, and contact with skin and eye . Use personal protective equipment, wear chemical impermeable gloves, and ensure adequate ventilation . In case of accidental ingestion or contact, seek immediate medical attention .
将来の方向性
While specific future directions for Methyl ricinoleate are not mentioned in the sources, it continues to be a subject of research in various fields, including its use in the production of fine chemicals and polymer precursors , and in the production of biodiesel from Ricinus communis seed oil .
Relevant Papers
作用機序
Target of Action
Methyl ricinoleate is a type of fatty acid methyl ester synthesized from castor oil and methyl alcohol . It is primarily used as a surfactant, cutting fluid additive, lubricant, and plasticizer . It serves as a plasticizer for cellulosic resins, polyvinyl acetate, and polystyrene . Therefore, its primary targets are these materials where it acts as a plasticizer.
Biochemical Pathways
It is known that it is a type of fatty acid methyl ester synthesized from castor oil and methyl alcohol . The synthesis process involves several reaction stages, including the Twitchell and Colgate-Emery processes, alkaline catalysis, transesterification with methyl ricinoleic, and lipase-catalyzed hydrolysis .
Result of Action
The primary result of methyl ricinoleate’s action is the increased flexibility and workability of the materials it is added to. This is due to its function as a plasticizer, which reduces the forces of attraction between polymer chains in materials such as cellulosic resins, polyvinyl acetate, and polystyrene .
特性
IUPAC Name |
methyl (Z,12R)-12-hydroxyoctadec-9-enoate | |
---|---|---|
Source | PubChem | |
URL | https://pubchem.ncbi.nlm.nih.gov | |
Description | Data deposited in or computed by PubChem | |
InChI |
InChI=1S/C19H36O3/c1-3-4-5-12-15-18(20)16-13-10-8-6-7-9-11-14-17-19(21)22-2/h10,13,18,20H,3-9,11-12,14-17H2,1-2H3/b13-10-/t18-/m1/s1 | |
Source | PubChem | |
URL | https://pubchem.ncbi.nlm.nih.gov | |
Description | Data deposited in or computed by PubChem | |
InChI Key |
XKGDWZQXVZSXAO-ADYSOMBNSA-N | |
Source | PubChem | |
URL | https://pubchem.ncbi.nlm.nih.gov | |
Description | Data deposited in or computed by PubChem | |
Canonical SMILES |
CCCCCCC(CC=CCCCCCCCC(=O)OC)O | |
Source | PubChem | |
URL | https://pubchem.ncbi.nlm.nih.gov | |
Description | Data deposited in or computed by PubChem | |
Isomeric SMILES |
CCCCCC[C@H](C/C=C\CCCCCCCC(=O)OC)O | |
Source | PubChem | |
URL | https://pubchem.ncbi.nlm.nih.gov | |
Description | Data deposited in or computed by PubChem | |
Molecular Formula |
C19H36O3 | |
Source | PubChem | |
URL | https://pubchem.ncbi.nlm.nih.gov | |
Description | Data deposited in or computed by PubChem | |
DSSTOX Substance ID |
DTXSID2029169 | |
Record name | Methyl ricinoleate | |
Source | EPA DSSTox | |
URL | https://comptox.epa.gov/dashboard/DTXSID2029169 | |
Description | DSSTox provides a high quality public chemistry resource for supporting improved predictive toxicology. | |
Molecular Weight |
312.5 g/mol | |
Source | PubChem | |
URL | https://pubchem.ncbi.nlm.nih.gov | |
Description | Data deposited in or computed by PubChem | |
Physical Description |
Liquid, Colorless liquid; [HSDB] | |
Record name | 9-Octadecenoic acid, 12-hydroxy-, methyl ester, (9Z,12R)- | |
Source | EPA Chemicals under the TSCA | |
URL | https://www.epa.gov/chemicals-under-tsca | |
Description | EPA Chemicals under the Toxic Substances Control Act (TSCA) collection contains information on chemicals and their regulations under TSCA, including non-confidential content from the TSCA Chemical Substance Inventory and Chemical Data Reporting. | |
Record name | Ricinoleic acid, methyl ester | |
Source | Haz-Map, Information on Hazardous Chemicals and Occupational Diseases | |
URL | https://haz-map.com/Agents/6965 | |
Description | Haz-Map® is an occupational health database designed for health and safety professionals and for consumers seeking information about the adverse effects of workplace exposures to chemical and biological agents. | |
Explanation | Copyright (c) 2022 Haz-Map(R). All rights reserved. Unless otherwise indicated, all materials from Haz-Map are copyrighted by Haz-Map(R). No part of these materials, either text or image may be used for any purpose other than for personal use. Therefore, reproduction, modification, storage in a retrieval system or retransmission, in any form or by any means, electronic, mechanical or otherwise, for reasons other than personal use, is strictly prohibited without prior written permission. | |
Boiling Point |
BP: 245 °C at 10 mm Hg | |
Record name | Ricinoleic acid, methyl ester | |
Source | Hazardous Substances Data Bank (HSDB) | |
URL | https://pubchem.ncbi.nlm.nih.gov/source/hsdb/5636 | |
Description | The Hazardous Substances Data Bank (HSDB) is a toxicology database that focuses on the toxicology of potentially hazardous chemicals. It provides information on human exposure, industrial hygiene, emergency handling procedures, environmental fate, regulatory requirements, nanomaterials, and related areas. The information in HSDB has been assessed by a Scientific Review Panel. | |
Solubility |
Soluble in alcohol, ether | |
Record name | Ricinoleic acid, methyl ester | |
Source | Hazardous Substances Data Bank (HSDB) | |
URL | https://pubchem.ncbi.nlm.nih.gov/source/hsdb/5636 | |
Description | The Hazardous Substances Data Bank (HSDB) is a toxicology database that focuses on the toxicology of potentially hazardous chemicals. It provides information on human exposure, industrial hygiene, emergency handling procedures, environmental fate, regulatory requirements, nanomaterials, and related areas. The information in HSDB has been assessed by a Scientific Review Panel. | |
Density |
0.9236 at 22 °C/4 °C | |
Record name | Ricinoleic acid, methyl ester | |
Source | Hazardous Substances Data Bank (HSDB) | |
URL | https://pubchem.ncbi.nlm.nih.gov/source/hsdb/5636 | |
Description | The Hazardous Substances Data Bank (HSDB) is a toxicology database that focuses on the toxicology of potentially hazardous chemicals. It provides information on human exposure, industrial hygiene, emergency handling procedures, environmental fate, regulatory requirements, nanomaterials, and related areas. The information in HSDB has been assessed by a Scientific Review Panel. | |
Color/Form |
Colorless liquid | |
CAS RN |
141-24-2 | |
Record name | Methyl ricinoleate | |
Source | CAS Common Chemistry | |
URL | https://commonchemistry.cas.org/detail?cas_rn=141-24-2 | |
Description | CAS Common Chemistry is an open community resource for accessing chemical information. Nearly 500,000 chemical substances from CAS REGISTRY cover areas of community interest, including common and frequently regulated chemicals, and those relevant to high school and undergraduate chemistry classes. This chemical information, curated by our expert scientists, is provided in alignment with our mission as a division of the American Chemical Society. | |
Explanation | The data from CAS Common Chemistry is provided under a CC-BY-NC 4.0 license, unless otherwise stated. | |
Record name | Ricinoleic acid, methyl ester | |
Source | ChemIDplus | |
URL | https://pubchem.ncbi.nlm.nih.gov/substance/?source=chemidplus&sourceid=0000141242 | |
Description | ChemIDplus is a free, web search system that provides access to the structure and nomenclature authority files used for the identification of chemical substances cited in National Library of Medicine (NLM) databases, including the TOXNET system. | |
Record name | Methyl ricinoleate | |
Source | DTP/NCI | |
URL | https://dtp.cancer.gov/dtpstandard/servlet/dwindex?searchtype=NSC&outputformat=html&searchlist=1254 | |
Description | The NCI Development Therapeutics Program (DTP) provides services and resources to the academic and private-sector research communities worldwide to facilitate the discovery and development of new cancer therapeutic agents. | |
Explanation | Unless otherwise indicated, all text within NCI products is free of copyright and may be reused without our permission. Credit the National Cancer Institute as the source. | |
Record name | 9-Octadecenoic acid, 12-hydroxy-, methyl ester, (9Z,12R)- | |
Source | EPA Chemicals under the TSCA | |
URL | https://www.epa.gov/chemicals-under-tsca | |
Description | EPA Chemicals under the Toxic Substances Control Act (TSCA) collection contains information on chemicals and their regulations under TSCA, including non-confidential content from the TSCA Chemical Substance Inventory and Chemical Data Reporting. | |
Record name | Methyl ricinoleate | |
Source | EPA DSSTox | |
URL | https://comptox.epa.gov/dashboard/DTXSID2029169 | |
Description | DSSTox provides a high quality public chemistry resource for supporting improved predictive toxicology. | |
Record name | Methyl ricinoleate | |
Source | European Chemicals Agency (ECHA) | |
URL | https://echa.europa.eu/substance-information/-/substanceinfo/100.004.976 | |
Description | The European Chemicals Agency (ECHA) is an agency of the European Union which is the driving force among regulatory authorities in implementing the EU's groundbreaking chemicals legislation for the benefit of human health and the environment as well as for innovation and competitiveness. | |
Explanation | Use of the information, documents and data from the ECHA website is subject to the terms and conditions of this Legal Notice, and subject to other binding limitations provided for under applicable law, the information, documents and data made available on the ECHA website may be reproduced, distributed and/or used, totally or in part, for non-commercial purposes provided that ECHA is acknowledged as the source: "Source: European Chemicals Agency, http://echa.europa.eu/". Such acknowledgement must be included in each copy of the material. ECHA permits and encourages organisations and individuals to create links to the ECHA website under the following cumulative conditions: Links can only be made to webpages that provide a link to the Legal Notice page. | |
Record name | METHYL RICINOLEATE | |
Source | FDA Global Substance Registration System (GSRS) | |
URL | https://gsrs.ncats.nih.gov/ginas/app/beta/substances/90FDR3O96Y | |
Description | The FDA Global Substance Registration System (GSRS) enables the efficient and accurate exchange of information on what substances are in regulated products. Instead of relying on names, which vary across regulatory domains, countries, and regions, the GSRS knowledge base makes it possible for substances to be defined by standardized, scientific descriptions. | |
Explanation | Unless otherwise noted, the contents of the FDA website (www.fda.gov), both text and graphics, are not copyrighted. They are in the public domain and may be republished, reprinted and otherwise used freely by anyone without the need to obtain permission from FDA. Credit to the U.S. Food and Drug Administration as the source is appreciated but not required. | |
Record name | Ricinoleic acid, methyl ester | |
Source | Hazardous Substances Data Bank (HSDB) | |
URL | https://pubchem.ncbi.nlm.nih.gov/source/hsdb/5636 | |
Description | The Hazardous Substances Data Bank (HSDB) is a toxicology database that focuses on the toxicology of potentially hazardous chemicals. It provides information on human exposure, industrial hygiene, emergency handling procedures, environmental fate, regulatory requirements, nanomaterials, and related areas. The information in HSDB has been assessed by a Scientific Review Panel. | |
Melting Point |
Freezing point: -4.5 °C | |
Record name | Ricinoleic acid, methyl ester | |
Source | Hazardous Substances Data Bank (HSDB) | |
URL | https://pubchem.ncbi.nlm.nih.gov/source/hsdb/5636 | |
Description | The Hazardous Substances Data Bank (HSDB) is a toxicology database that focuses on the toxicology of potentially hazardous chemicals. It provides information on human exposure, industrial hygiene, emergency handling procedures, environmental fate, regulatory requirements, nanomaterials, and related areas. The information in HSDB has been assessed by a Scientific Review Panel. | |
Retrosynthesis Analysis
AI-Powered Synthesis Planning: Our tool employs the Template_relevance Pistachio, Template_relevance Bkms_metabolic, Template_relevance Pistachio_ringbreaker, Template_relevance Reaxys, Template_relevance Reaxys_biocatalysis model, leveraging a vast database of chemical reactions to predict feasible synthetic routes.
One-Step Synthesis Focus: Specifically designed for one-step synthesis, it provides concise and direct routes for your target compounds, streamlining the synthesis process.
Accurate Predictions: Utilizing the extensive PISTACHIO, BKMS_METABOLIC, PISTACHIO_RINGBREAKER, REAXYS, REAXYS_BIOCATALYSIS database, our tool offers high-accuracy predictions, reflecting the latest in chemical research and data.
Strategy Settings
Precursor scoring | Relevance Heuristic |
---|---|
Min. plausibility | 0.01 |
Model | Template_relevance |
Template Set | Pistachio/Bkms_metabolic/Pistachio_ringbreaker/Reaxys/Reaxys_biocatalysis |
Top-N result to add to graph | 6 |
Feasible Synthetic Routes
Q & A
Q1: What is the molecular formula and weight of methyl ricinoleate?
A1: Methyl ricinoleate has a molecular formula of C19H36O3 and a molecular weight of 312.49 g/mol. []
Q2: How can the structure of methyl ricinoleate be confirmed?
A2: Spectroscopic techniques like Fourier Transform Infrared (FTIR) spectroscopy, Nuclear Magnetic Resonance (NMR) spectroscopy (both 1H and 13C), and Gas Chromatography-Mass Spectrometry (GC-MS) are commonly used to confirm the structure of methyl ricinoleate. [, , , , , , , ]
Q3: What is the solubility of methyl ricinoleate in common solvents?
A3: Methyl ricinoleate is miscible with diesel fuel and soluble in various organic solvents, including toluene, hexane, and diethyl ether. [, ]
Q4: What is the thermal stability of methyl ricinoleate?
A4: The thermal stability of methyl ricinoleate can vary depending on the specific application and conditions. Studies have shown that it can undergo pyrolysis at temperatures above 500°C, leading to the formation of various products like undecylenic acid methyl ester and heptanal. [, ]
Q5: How does the presence of a hydroxyl group in methyl ricinoleate influence its properties?
A5: The hydroxyl group in methyl ricinoleate makes it amenable to various chemical modifications like etherification, esterification, and amidation, allowing the synthesis of derivatives with tailored properties. [, , ]
Q6: Can methyl ricinoleate be used as a starting material for biodiesel production?
A6: Yes, methyl ricinoleate, often obtained from transesterification of castor oil, can be further converted into biodiesel through transesterification reactions using suitable catalysts. [, ]
Q7: What are some notable applications of methyl ricinoleate in the chemical industry?
A8: Methyl ricinoleate serves as a valuable platform chemical for synthesizing various compounds like polymers, surfactants, and biolubricants. Its unique structure, featuring a hydroxyl group and a double bond, allows for diverse chemical transformations. [, , , , ]
Q8: Have computational methods been used to study methyl ricinoleate?
A9: Yes, Density Functional Theory (DFT) calculations have been employed to study the pyrolysis mechanism of methyl ricinoleate, providing insights into the bond dissociation energies and activation energies associated with the formation of different products. []
Q9: How does the position of the double bond in methyl ricinoleate impact its reactivity?
A10: The position of the double bond in methyl ricinoleate influences its reactivity in reactions like epoxidation and metathesis. [, ]
Q10: How does the presence of the hydroxyl group in methyl ricinoleate influence its reactivity?
A11: The hydroxyl group in methyl ricinoleate can participate in various reactions like esterification, etherification, and amidation, enabling the synthesis of a wide range of derivatives with diverse properties. [, , ]
Q11: How can the stability of methyl ricinoleate be improved?
A12: The stability of methyl ricinoleate can be enhanced by using antioxidants, storing it under inert atmospheres, and avoiding exposure to light and heat. []
Q12: What analytical methods are commonly used to quantify methyl ricinoleate?
A13: Gas Chromatography (GC), often coupled with a Flame Ionization Detector (FID) or a Mass Spectrometer (MS), is widely employed to quantify methyl ricinoleate in various matrices. [, ]
Q13: What factors can influence the dissolution rate of methyl ricinoleate?
A15: Factors like temperature, agitation speed, and the nature of the solvent or medium can influence the dissolution rate of methyl ricinoleate. []
試験管内研究製品の免責事項と情報
BenchChemで提示されるすべての記事および製品情報は、情報提供を目的としています。BenchChemで購入可能な製品は、生体外研究のために特別に設計されています。生体外研究は、ラテン語の "in glass" に由来し、生物体の外で行われる実験を指します。これらの製品は医薬品または薬として分類されておらず、FDAから任何の医療状態、病気、または疾患の予防、治療、または治癒のために承認されていません。これらの製品を人間または動物に体内に導入する形態は、法律により厳格に禁止されています。これらのガイドラインに従うことは、研究と実験において法的および倫理的な基準の遵守を確実にするために重要です。