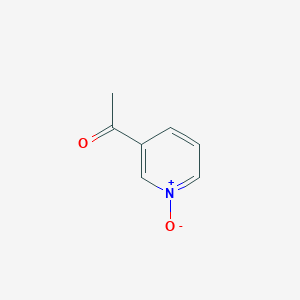
3-Acetylpyridine N-oxide
概要
説明
3-Acetylpyridine N-oxide is a compound with the molecular formula C7H7NO2 and a molecular weight of 137.14 . It is also known by other names such as 1-(1-oxidopyridin-3-yl)ethanone .
Synthesis Analysis
The synthesis of 3-Acetylpyridine N-oxide has been described in various studies. One process involves the reaction of a pyridinecarboxylic ester with acetic acid in the gas phase in the presence of a catalyst . Another method involves the C2-functionalization of pyridine and quinoline N-oxides .Molecular Structure Analysis
The molecular structure of 3-Acetylpyridine N-oxide consists of 10 heavy atoms . The InChI code for the compound is 1S/C7H7NO2/c1-6(9)7-3-2-4-8(10)5-7/h2-5H,1H3 . The compound has a topological polar surface area of 42.5 Ų .Chemical Reactions Analysis
3-Acetylpyridine N-oxide can undergo various chemical reactions. For instance, it can be involved in the direct C−H-functionalization of azines . Another reaction involves the nitration of pyridine compounds .Physical And Chemical Properties Analysis
3-Acetylpyridine N-oxide is a solid at room temperature . It has a computed XLogP3 value of -0.6, indicating its solubility in water and other polar solvents . The compound has no hydrogen bond donors and two hydrogen bond acceptors .科学的研究の応用
Asymmetric Synthesis
3-Acetylpyridine N-oxide: has been utilized in asymmetric synthesis, particularly in catalytic asymmetric formal [3 + 3] annulation reactions . This process involves the combination of 2-enoyl-pyridine N-oxide with ketones to produce cyclohexene derivatives. The presence of the N-oxide allows for the use of chiral organocatalysts, leading to enantioselective outcomes. This is significant in the production of chiral molecules that are prevalent in pharmaceuticals.
Biological Activity Profiling
Compounds containing the 3-Acetylpyridine N-oxide motif have been identified to possess specific biological activities . These activities include potential therapeutic effects, making them candidates for drug development. The N-oxide group contributes to the molecule’s bioactivity by influencing its interaction with biological targets.
Personal Care Products
The unique properties of 3-Acetylpyridine N-oxide derivatives make them suitable for inclusion in personal care products . Their structural motif can be found in ingredients used in cosmetics, detergents, and hygiene products, where they contribute to the stability and efficacy of the product.
Oxidation Reactions
3-Acetylpyridine N-oxide: serves as an oxidant in organic reactions . Its ability to transfer oxygen atoms can be harnessed in various synthetic pathways, facilitating the transformation of substrates into more complex molecules.
Chiral Ligands and Organocatalysts
The enantiomerically enriched form of 3-Acetylpyridine N-oxide derivatives are used as chiral ligands or organocatalysts in asymmetric synthesis . They play a crucial role in inducing chirality in the synthesized products, which is vital for the activity of many pharmaceuticals.
Lipid C═C Location-Resolved MS Imaging
A novel application of 3-Acetylpyridine N-oxide is in lipid C═C location-resolved mass spectrometry (MS) imaging . It is used in on-tissue charge-switching Paternò–Büchi derivatization, enabling the detection and assignment of C═C locations in glycerophospholipids and neutral lipids. This has profound implications for studying the spatial distribution of lipid isomers in biological tissues and their association with diseases.
Neurodegenerative Disease Research
The same MS imaging technique mentioned above has been applied to map the spatial distribution of lipid C═C location isomers in Alzheimer’s disease (AD) mouse models . This application provides insights into the relationship between lipid structural diversity and neurodegenerative diseases, potentially leading to new biomarkers or therapeutic targets.
Environmental Analysis
3-Acetylpyridine N-oxide: can be used in environmental analysis to study the presence and behavior of nitrogen oxides in various ecosystems . Its reactivity and stability under environmental conditions make it a useful tool for tracing and quantifying nitrogen-related processes.
Safety And Hazards
3-Acetylpyridine N-oxide is considered hazardous according to the 2012 OSHA Hazard Communication Standard . It is toxic if swallowed and can cause skin and eye irritation . Safety measures include washing thoroughly after handling, wearing protective clothing, and seeking medical attention if irritation persists .
将来の方向性
While specific future directions for 3-Acetylpyridine N-oxide were not found in the retrieved papers, heterocyclic N-oxides are emerging as potent compounds with various therapeutic activities . This suggests potential future research directions in exploring the biological activities of 3-Acetylpyridine N-oxide and its derivatives.
特性
IUPAC Name |
1-(1-oxidopyridin-1-ium-3-yl)ethanone | |
---|---|---|
Source | PubChem | |
URL | https://pubchem.ncbi.nlm.nih.gov | |
Description | Data deposited in or computed by PubChem | |
InChI |
InChI=1S/C7H7NO2/c1-6(9)7-3-2-4-8(10)5-7/h2-5H,1H3 | |
Source | PubChem | |
URL | https://pubchem.ncbi.nlm.nih.gov | |
Description | Data deposited in or computed by PubChem | |
InChI Key |
AZFMFGIMDGBJRR-UHFFFAOYSA-N | |
Source | PubChem | |
URL | https://pubchem.ncbi.nlm.nih.gov | |
Description | Data deposited in or computed by PubChem | |
Canonical SMILES |
CC(=O)C1=C[N+](=CC=C1)[O-] | |
Source | PubChem | |
URL | https://pubchem.ncbi.nlm.nih.gov | |
Description | Data deposited in or computed by PubChem | |
Molecular Formula |
C7H7NO2 | |
Source | PubChem | |
URL | https://pubchem.ncbi.nlm.nih.gov | |
Description | Data deposited in or computed by PubChem | |
DSSTOX Substance ID |
DTXSID60161843 | |
Record name | 3-Acetylpyridine N-oxide | |
Source | EPA DSSTox | |
URL | https://comptox.epa.gov/dashboard/DTXSID60161843 | |
Description | DSSTox provides a high quality public chemistry resource for supporting improved predictive toxicology. | |
Molecular Weight |
137.14 g/mol | |
Source | PubChem | |
URL | https://pubchem.ncbi.nlm.nih.gov | |
Description | Data deposited in or computed by PubChem | |
Product Name |
3-Acetylpyridine N-oxide | |
CAS RN |
14188-94-4 | |
Record name | 1-(1-Oxido-3-pyridinyl)ethanone | |
Source | CAS Common Chemistry | |
URL | https://commonchemistry.cas.org/detail?cas_rn=14188-94-4 | |
Description | CAS Common Chemistry is an open community resource for accessing chemical information. Nearly 500,000 chemical substances from CAS REGISTRY cover areas of community interest, including common and frequently regulated chemicals, and those relevant to high school and undergraduate chemistry classes. This chemical information, curated by our expert scientists, is provided in alignment with our mission as a division of the American Chemical Society. | |
Explanation | The data from CAS Common Chemistry is provided under a CC-BY-NC 4.0 license, unless otherwise stated. | |
Record name | 3-Acetylpyridine N-oxide | |
Source | ChemIDplus | |
URL | https://pubchem.ncbi.nlm.nih.gov/substance/?source=chemidplus&sourceid=0014188944 | |
Description | ChemIDplus is a free, web search system that provides access to the structure and nomenclature authority files used for the identification of chemical substances cited in National Library of Medicine (NLM) databases, including the TOXNET system. | |
Record name | 3-Acetylpyridine N-oxide | |
Source | EPA DSSTox | |
URL | https://comptox.epa.gov/dashboard/DTXSID60161843 | |
Description | DSSTox provides a high quality public chemistry resource for supporting improved predictive toxicology. | |
Synthesis routes and methods
Procedure details
Retrosynthesis Analysis
AI-Powered Synthesis Planning: Our tool employs the Template_relevance Pistachio, Template_relevance Bkms_metabolic, Template_relevance Pistachio_ringbreaker, Template_relevance Reaxys, Template_relevance Reaxys_biocatalysis model, leveraging a vast database of chemical reactions to predict feasible synthetic routes.
One-Step Synthesis Focus: Specifically designed for one-step synthesis, it provides concise and direct routes for your target compounds, streamlining the synthesis process.
Accurate Predictions: Utilizing the extensive PISTACHIO, BKMS_METABOLIC, PISTACHIO_RINGBREAKER, REAXYS, REAXYS_BIOCATALYSIS database, our tool offers high-accuracy predictions, reflecting the latest in chemical research and data.
Strategy Settings
Precursor scoring | Relevance Heuristic |
---|---|
Min. plausibility | 0.01 |
Model | Template_relevance |
Template Set | Pistachio/Bkms_metabolic/Pistachio_ringbreaker/Reaxys/Reaxys_biocatalysis |
Top-N result to add to graph | 6 |
Feasible Synthetic Routes
Q & A
Q1: What is the metabolic fate of 3-acetylpyridine N-oxide in liver tissue?
A1: Research indicates that 3-acetylpyridine N-oxide undergoes keto-reduction when incubated with liver tissue fractions (both soluble and 10,000 g fractions). This metabolic transformation primarily yields 1-(3-pyridyl-N-oxide)ethanol. [] Interestingly, while trace amounts of 3-acetylpyridine are also formed, suggesting N-oxide reduction, this pathway seems less prominent. []
試験管内研究製品の免責事項と情報
BenchChemで提示されるすべての記事および製品情報は、情報提供を目的としています。BenchChemで購入可能な製品は、生体外研究のために特別に設計されています。生体外研究は、ラテン語の "in glass" に由来し、生物体の外で行われる実験を指します。これらの製品は医薬品または薬として分類されておらず、FDAから任何の医療状態、病気、または疾患の予防、治療、または治癒のために承認されていません。これらの製品を人間または動物に体内に導入する形態は、法律により厳格に禁止されています。これらのガイドラインに従うことは、研究と実験において法的および倫理的な基準の遵守を確実にするために重要です。