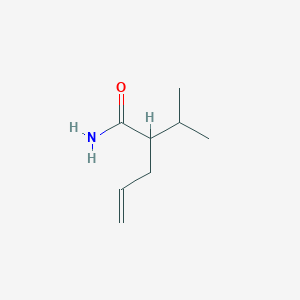
アリルイソプロピルアセトアミド
概要
説明
Allylisopropylacetamide is an organic compound with the molecular formula C8H15NO. It is a colorless to pale yellow liquid with a pungent odor. This compound is soluble in water and most organic solvents. Allylisopropylacetamide is known for its role as a porphyrogenic agent, meaning it can induce the production of porphyrins, which are precursors to heme, an essential component of hemoglobin.
科学的研究の応用
Biochemical Effects
Allylisopropylacetamide is known primarily as a porphyrogenic drug, which means it can induce the synthesis of porphyrins in the body. Key studies have demonstrated its significant impact on liver biochemistry:
- Induction of Delta-Aminolevulinate Synthetase : This compound is a potent inducer of delta-aminolevulinate synthetase, an enzyme critical for heme biosynthesis. Research indicates that allylisopropylacetamide specifically increases nucleoplasmic RNA synthesis in rat liver, suggesting its role in enhancing gene expression related to heme production .
- Microsomal Protein Synthesis : Successive administrations of allylisopropylacetamide have been shown to increase liver weight and microsomal protein content. It enhances the rate of microsomal protein synthesis both in vivo and in vitro, while decreasing ribonuclease activity and increasing NADPH-cytochrome c reductase activity .
- Effects on Cytochrome P450 : Unlike phenobarbital, another well-known inducer of heme synthesis, allylisopropylacetamide causes an initial decrease in cytochrome P450 content, which is significant for understanding its unique biochemical pathway .
Experimental Applications
Allylisopropylacetamide has been utilized in various experimental settings to study liver function and porphyria:
- Porphyrogenic Studies : It serves as a model compound to investigate the mechanisms of porphyria, a group of disorders caused by the accumulation of porphyrins due to enzyme deficiencies. Its administration in animal models helps elucidate the biochemical pathways involved in porphyrin metabolism .
- Hepatic Function Research : The compound's ability to induce changes in liver weight and enzyme activity makes it valuable for studying hepatic function and drug metabolism. It allows researchers to explore the effects of different substances on liver physiology and pathology .
Therapeutic Implications
While primarily used for research purposes, the effects of allylisopropylacetamide on liver enzymes and porphyrin synthesis may have therapeutic implications:
- Potential Treatment for Porphyrias : Understanding how allylisopropylacetamide induces porphyrin synthesis could lead to novel therapeutic strategies for treating certain types of porphyrias by modulating enzyme activity or compensating for deficiencies .
- Drug Interaction Studies : Its effects on cytochrome P450 enzymes make it a candidate for studying drug interactions and metabolism, which is crucial for developing safer pharmacological agents .
Case Studies and Research Findings
Several studies have highlighted the applications and effects of allylisopropylacetamide:
作用機序
Target of Action
Allylisopropylacetamide (AlA) primarily targets the liver, specifically the hepatic cells . It interacts with the cytochrome P450-dependent mixed function oxidase (MFO) systems, which are crucial for the metabolism of drugs and environmental xenobiotics .
Mode of Action
AlA is known to be a “suicide inhibitor” of cytochrome P450 . It is first oxidized by the cytochrome to a reactive intermediate that binds covalently to the heme moiety of the cytochrome . The subsequent breakdown of the heme gives rise to characteristic pigments .
Biochemical Pathways
AlA affects the heme biosynthetic pathway. It induces an increase in the synthesis of porphobilinogen (PBG), a precursor in the heme biosynthetic pathway . This increase could be due to an increased synthesis of PBG or a decreased utilization . PBG is synthesized in the liver via 8-aminolevulinic acid (ALA) from glycine and acetate or succinate .
Pharmacokinetics
Multiple intraperitoneal injections of AlA in rats cause a decrease in matrix material and loss of endogenous peroxidatic activity in hepatic cell microbodies . These changes are detectable as early as 48 hours after commencing injections and are prominent by 5 days . On cessation of injections, recovery of normal microbody morphology is rapid but peroxidatic activity of microbodies returns more slowly .
Result of Action
The administration of AlA results in porphobilinogenuria in rabbits . In rats, it causes a decrease in matrix material and loss of endogenous peroxidatic activity in hepatic cell microbodies . These changes are detectable as early as 48 hours after commencing injections and are prominent by 5 days .
Action Environment
Environmental factors, including other chemicals and xenobiotics, can influence the action of AlA. For instance, most chemicals either induce or have little or no effect on MFO systems, but a few, including AlA, produce marked losses . Other agents like carbon tetrachloride and phenobarbital, which also affect the MFO systems, can interact with AlA, potentially influencing its efficacy and stability .
生化学分析
Biochemical Properties
Allylisopropylacetamide plays a significant role in biochemical reactions, particularly in the induction of delta-aminolevulinate synthetase, an enzyme crucial for heme biosynthesis . The compound interacts with various enzymes and proteins, including cytochrome P-450 and NADPH-cytochrome c reductase . These interactions lead to an increase in the rate of microsomal protein synthesis and a decrease in microsomal ribonuclease activity . Allylisopropylacetamide also causes an initial decrease in cytochrome P-450 content, which is not observed with other compounds like phenobarbital .
Cellular Effects
The effects of Allylisopropylacetamide on cellular processes are profound. In hepatic cells, multiple intraperitoneal injections of Allylisopropylacetamide cause a decrease in matrix material and loss of endogenous peroxidatic activity in microbodies . These changes are detectable as early as 48 hours after commencing injections and become prominent by 5 days . The compound also influences cell signaling pathways and gene expression, particularly in the context of heme biosynthesis and cytochrome P-450 levels .
Molecular Mechanism
At the molecular level, Allylisopropylacetamide exerts its effects by inducing the activity of delta-aminolevulinate synthetase in liver mitochondria . This induction is greatly suppressed by the administration of hemin, hemoglobin, or bilirubin . The compound also affects the activity of NADPH cytochrome P-450 reductase, leading to changes in cytochrome P-450 levels in both mitochondrial and microsomal fractions . These interactions highlight the compound’s role in modulating enzyme activity and gene expression related to heme biosynthesis.
Temporal Effects in Laboratory Settings
The temporal effects of Allylisopropylacetamide in laboratory settings have been well-documented. Successive administrations of the compound increase liver weight, microsomal protein, and phospholipid contents . The rate of microsomal protein synthesis increases both in vivo and in vitro . The compound also causes a decrease in microsomal ribonuclease activity and an initial decrease in cytochrome P-450 content . These effects are reversible upon cessation of injections, with recovery of normal microbody morphology occurring rapidly, although peroxidatic activity returns more slowly .
Dosage Effects in Animal Models
In animal models, the effects of Allylisopropylacetamide vary with different dosages. High doses of the compound can lead to toxic effects, including significant alterations in liver function and enzyme activity . For instance, chronic administration of Allylisopropylacetamide diminishes both mitochondrial and microsomal cytochrome P-450 brain levels . These dosage-dependent effects highlight the importance of careful dosage regulation in experimental settings.
Metabolic Pathways
Allylisopropylacetamide is involved in several metabolic pathways, particularly those related to heme biosynthesis. The compound induces delta-aminolevulinate synthetase, the first and rate-limiting enzyme of the heme biosynthetic pathway . This induction leads to increased heme and cytochrome P-450 synthesis, enhancing the activity of the mixed-function oxidase system . The compound also interacts with various enzymes and cofactors involved in these pathways, further influencing metabolic flux and metabolite levels .
Transport and Distribution
The transport and distribution of Allylisopropylacetamide within cells and tissues involve several key processes. The compound is known to inhibit the de novo formation of catalase in guinea pig liver parenchymal cells . It is transported from peroxisomes into the cytoplasm, where it is subsequently degraded . These transport mechanisms are crucial for understanding the compound’s overall distribution and localization within cells.
Subcellular Localization
Allylisopropylacetamide exhibits specific subcellular localization patterns, particularly in hepatic cells. The compound causes a decrease in matrix material and loss of endogenous peroxidatic activity in microbodies . These changes are detectable as early as 48 hours after commencing injections and become prominent by 5 days . The subcellular localization of Allylisopropylacetamide is influenced by various targeting signals and post-translational modifications, which direct it to specific compartments or organelles within the cell .
準備方法
Synthetic Routes and Reaction Conditions: Allylisopropylacetamide can be synthesized through various methods. One common synthetic route involves the reaction of allyl bromide with isopropylamine to form allylisopropylamine. This intermediate is then reacted with acetic anhydride to yield allylisopropylacetamide. The reaction conditions typically involve the use of a solvent such as methanol and a catalyst to facilitate the reaction.
Industrial Production Methods: In an industrial setting, the production of allylisopropylacetamide may involve continuous flow reactors to ensure consistent product quality and yield. The process may also include purification steps such as distillation or recrystallization to obtain the desired purity of the compound.
化学反応の分析
Types of Reactions: Allylisopropylacetamide undergoes various chemical reactions, including:
Oxidation: It can be oxidized to form corresponding oxides or other oxidation products.
Reduction: Reduction reactions can convert it into different amine derivatives.
Substitution: Allylisopropylacetamide can participate in substitution reactions where functional groups are replaced by other groups.
Common Reagents and Conditions:
Oxidation: Common oxidizing agents include potassium permanganate and hydrogen peroxide.
Reduction: Reducing agents such as lithium aluminum hydride or sodium borohydride are often used.
Substitution: Halogenating agents like bromine or chlorine can be used for substitution reactions.
Major Products: The major products formed from these reactions depend on the specific reagents and conditions used. For example, oxidation may yield oxides, while reduction may produce amine derivatives.
類似化合物との比較
Allylisopropylacetamide: Known for its role as a porphyrogenic agent.
Aminolevulinic Acid: Another compound involved in heme synthesis, but it acts as a precursor rather than an inhibitor.
Succinylacetone: An inhibitor of heme synthesis, similar to allylisopropylacetamide, but with different molecular targets.
Uniqueness: Allylisopropylacetamide is unique in its ability to induce porphyria by inhibiting cytochrome P450 enzymes, leading to the accumulation of porphyrin precursors. This property makes it a valuable tool for studying the biochemical pathways involved in heme synthesis and the effects of porphyrogenic agents on liver function.
生物活性
Allylisopropylacetamide (AIA) is a compound that has garnered attention in pharmacological and toxicological research due to its complex biological activity, particularly its effects on liver metabolism and enzyme induction. This article synthesizes findings from various studies to provide a comprehensive overview of AIA's biological activity, including its mechanisms of action, effects on liver enzymes, and implications for porphyria.
Allylisopropylacetamide is known to influence several metabolic pathways in the liver. One of the primary mechanisms involves the regulation of δ-aminolevulinic acid (ALA) synthetase , an enzyme crucial for heme synthesis. Studies have shown that AIA administration can lead to increased activity of ALA synthetase, resulting in elevated porphyrin levels in plasma. This effect is particularly significant as it can contribute to the development of porphyria, a group of disorders characterized by the accumulation of porphyrins due to disrupted heme synthesis .
Enzyme Induction and Inhibition
AIA has been reported to induce certain liver enzymes while inhibiting others. For instance:
- Induction of ALA Synthetase : As mentioned, AIA increases the activity of ALA synthetase, which is involved in heme production .
- Inhibition of Drug-Metabolizing Enzymes : Research indicates that AIA can inhibit drug-metabolizing enzymes, particularly after treatment with phenobarbital (PB). This inhibition results in reduced clearance rates for drugs like antipyrine, which could have significant implications for drug metabolism and efficacy .
The following table summarizes key findings related to AIA's effects on liver enzymes:
Enzyme | Effect | Study Reference |
---|---|---|
δ-Aminolevulinic Acid Synthetase | Increased activity | |
Catalase | Decreased activity | |
Drug-Metabolizing Enzymes | Inhibited |
Case Studies and Research Findings
Several studies have illustrated the impact of AIA on hepatic functions:
- Porphyrin Accumulation : In a study involving rats, administration of AIA resulted in significant increases in porphyrin levels, indicating its role in inducing porphyria. The study highlighted the protective effects against porphyrin accumulation when certain antioxidants were administered alongside AIA .
- Hepatic Enzyme Activity Alterations : Research conducted on C57BL/6J male mice demonstrated that AIA treatment led to a decline in hepatic catalase activity, suggesting potential oxidative stress implications due to altered enzyme profiles .
- Comparative Studies : Investigations into the effects of AIA on young versus adult rat livers revealed that while it does not induce enzyme degradation in younger rats, it significantly alters enzyme activities in adults, pointing towards developmental differences in metabolic responses to xenobiotics .
Implications for Toxicology
The dual nature of AIA as both an inducer and inhibitor of various enzymes poses important considerations for toxicology. Its ability to induce porphyria highlights the need for careful monitoring when used therapeutically or encountered as an environmental contaminant. Furthermore, understanding its metabolic pathways can aid in predicting interactions with other drugs and potential adverse effects.
特性
IUPAC Name |
2-propan-2-ylpent-4-enamide | |
---|---|---|
Source | PubChem | |
URL | https://pubchem.ncbi.nlm.nih.gov | |
Description | Data deposited in or computed by PubChem | |
InChI |
InChI=1S/C8H15NO/c1-4-5-7(6(2)3)8(9)10/h4,6-7H,1,5H2,2-3H3,(H2,9,10) | |
Source | PubChem | |
URL | https://pubchem.ncbi.nlm.nih.gov | |
Description | Data deposited in or computed by PubChem | |
InChI Key |
HINLFAQOKAHXOD-UHFFFAOYSA-N | |
Source | PubChem | |
URL | https://pubchem.ncbi.nlm.nih.gov | |
Description | Data deposited in or computed by PubChem | |
Canonical SMILES |
CC(C)C(CC=C)C(=O)N | |
Source | PubChem | |
URL | https://pubchem.ncbi.nlm.nih.gov | |
Description | Data deposited in or computed by PubChem | |
Molecular Formula |
C8H15NO | |
Source | PubChem | |
URL | https://pubchem.ncbi.nlm.nih.gov | |
Description | Data deposited in or computed by PubChem | |
DSSTOX Substance ID |
DTXSID20952350 | |
Record name | 2-(Propan-2-yl)pent-4-enimidic acid | |
Source | EPA DSSTox | |
URL | https://comptox.epa.gov/dashboard/DTXSID20952350 | |
Description | DSSTox provides a high quality public chemistry resource for supporting improved predictive toxicology. | |
Molecular Weight |
141.21 g/mol | |
Source | PubChem | |
URL | https://pubchem.ncbi.nlm.nih.gov | |
Description | Data deposited in or computed by PubChem | |
CAS No. |
299-78-5 | |
Record name | 2-(1-Methylethyl)-4-pentenamide | |
Source | CAS Common Chemistry | |
URL | https://commonchemistry.cas.org/detail?cas_rn=299-78-5 | |
Description | CAS Common Chemistry is an open community resource for accessing chemical information. Nearly 500,000 chemical substances from CAS REGISTRY cover areas of community interest, including common and frequently regulated chemicals, and those relevant to high school and undergraduate chemistry classes. This chemical information, curated by our expert scientists, is provided in alignment with our mission as a division of the American Chemical Society. | |
Explanation | The data from CAS Common Chemistry is provided under a CC-BY-NC 4.0 license, unless otherwise stated. | |
Record name | Allylisopropylacetamide | |
Source | ChemIDplus | |
URL | https://pubchem.ncbi.nlm.nih.gov/substance/?source=chemidplus&sourceid=0000299785 | |
Description | ChemIDplus is a free, web search system that provides access to the structure and nomenclature authority files used for the identification of chemical substances cited in National Library of Medicine (NLM) databases, including the TOXNET system. | |
Record name | 2-(Propan-2-yl)pent-4-enimidic acid | |
Source | EPA DSSTox | |
URL | https://comptox.epa.gov/dashboard/DTXSID20952350 | |
Description | DSSTox provides a high quality public chemistry resource for supporting improved predictive toxicology. | |
Record name | 2-ISOPROPYL-4-PENTENAMIDE | |
Source | FDA Global Substance Registration System (GSRS) | |
URL | https://gsrs.ncats.nih.gov/ginas/app/beta/substances/ZOF4719QS5 | |
Description | The FDA Global Substance Registration System (GSRS) enables the efficient and accurate exchange of information on what substances are in regulated products. Instead of relying on names, which vary across regulatory domains, countries, and regions, the GSRS knowledge base makes it possible for substances to be defined by standardized, scientific descriptions. | |
Explanation | Unless otherwise noted, the contents of the FDA website (www.fda.gov), both text and graphics, are not copyrighted. They are in the public domain and may be republished, reprinted and otherwise used freely by anyone without the need to obtain permission from FDA. Credit to the U.S. Food and Drug Administration as the source is appreciated but not required. | |
Retrosynthesis Analysis
AI-Powered Synthesis Planning: Our tool employs the Template_relevance Pistachio, Template_relevance Bkms_metabolic, Template_relevance Pistachio_ringbreaker, Template_relevance Reaxys, Template_relevance Reaxys_biocatalysis model, leveraging a vast database of chemical reactions to predict feasible synthetic routes.
One-Step Synthesis Focus: Specifically designed for one-step synthesis, it provides concise and direct routes for your target compounds, streamlining the synthesis process.
Accurate Predictions: Utilizing the extensive PISTACHIO, BKMS_METABOLIC, PISTACHIO_RINGBREAKER, REAXYS, REAXYS_BIOCATALYSIS database, our tool offers high-accuracy predictions, reflecting the latest in chemical research and data.
Strategy Settings
Precursor scoring | Relevance Heuristic |
---|---|
Min. plausibility | 0.01 |
Model | Template_relevance |
Template Set | Pistachio/Bkms_metabolic/Pistachio_ringbreaker/Reaxys/Reaxys_biocatalysis |
Top-N result to add to graph | 6 |
Feasible Synthetic Routes
試験管内研究製品の免責事項と情報
BenchChemで提示されるすべての記事および製品情報は、情報提供を目的としています。BenchChemで購入可能な製品は、生体外研究のために特別に設計されています。生体外研究は、ラテン語の "in glass" に由来し、生物体の外で行われる実験を指します。これらの製品は医薬品または薬として分類されておらず、FDAから任何の医療状態、病気、または疾患の予防、治療、または治癒のために承認されていません。これらの製品を人間または動物に体内に導入する形態は、法律により厳格に禁止されています。これらのガイドラインに従うことは、研究と実験において法的および倫理的な基準の遵守を確実にするために重要です。