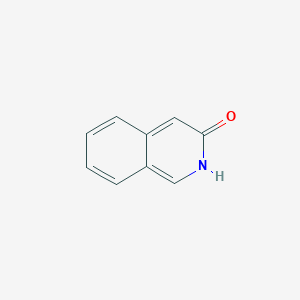
3-Hydroxyisoquinoline
概要
説明
3-Hydroxyisoquinoline is a compound used in a polymeric mixture of polyvinyl alcohol (PVA) along with Nile Red to produce white light through Förster resonance energy transfer . It is a natural product found in Ruta montana .
Synthesis Analysis
A convenient method for the synthesis of 3-hydroxyisoquinolines from β-ketoesters using a one-pot aryne acyl-alkylation/condensation procedure has been disclosed .
Molecular Structure Analysis
The molecular structure analysis of 3-Hydroxyisoquinoline is complex and involves various factors. More detailed information can be found in the referenced literature .
Chemical Reactions Analysis
Two excited state proton transfer mechanisms of 3-hydroxyisoquinoline (3HIQ) in cyclohexane and acetic acid have been investigated based on the time-dependent density functional theory (TDDFT), suggesting a different double-proton transfer mechanism from the one proposed previously .
Physical And Chemical Properties Analysis
The physical and chemical properties of 3-Hydroxyisoquinoline are diverse and depend on the conditions. Spectral and photophysical properties of 3-HIQ in various protic/aprotic solvents have been studied .
科学的研究の応用
Photophysical Studies: Proton Transfer Mechanisms
The compound’s photophysical properties are of interest in scientific research. Studies have investigated two excited state proton transfer mechanisms of 3-Hydroxyisoquinoline in solvents like cyclohexane and acetic acid using time-dependent density functional theory (TDDFT) . These findings are significant for understanding the behavior of organic molecules under various conditions.
Spectroscopy: Solvent Interaction Analysis
3-Hydroxyisoquinoline: is also studied for its spectral properties in different protic and aprotic solvents. The way it interacts with solvents can reveal information about solvent polarity and the solute’s electronic structure. This is crucial for applications in spectroscopy where understanding these interactions is key .
Photochemistry: Phototautomerization
The compound exhibits phototautomerization, a process where a molecule in its excited state undergoes a structural rearrangement. This property of 3-Hydroxyisoquinoline is studied to understand the dynamics of photochemical reactions, which has implications in fields like material sciences and photodynamic therapy .
Chemical Biology: Nucleophilic Attack Studies
In chemical biology, 3-Hydroxyisoquinoline is used to study nucleophilic attack mechanisms. Research has shown that, depending on the nucleophile, an attack can occur at different positions on the heterocyclic substrate, leading to various reaction products. This is important for understanding reaction pathways in biological systems .
作用機序
Target of Action
The primary target of 3-Hydroxyisoquinoline (3HIQ) is the proton transfer mechanisms in the excited state . This compound is involved in a double-proton transfer mechanism, which is a key process in many biological systems .
Mode of Action
3HIQ interacts with its targets through a process known as excited state proton transfer . This involves the transfer of protons in the excited state, which is a fundamental process in photochemistry and photobiology . The compound exists in two forms: the normal form (enol/enol) and the tautomer form (keto/keto) in cyclohexane . Upon optical excitation, the enol/enol form is excited to the first excited state, then transfers one proton, in turn, transition to the ground state to transfer another proton .
Biochemical Pathways
The biochemical pathways affected by 3HIQ involve the excited state proton transfer mechanisms . The compound’s interaction with these pathways results in phototautomerization, a process that can take place in the excited state through excited state intramolecular proton . This process is controlled by the polarity of the solvent .
Pharmacokinetics
The compound’s excited state proton transfer mechanisms suggest that its bioavailability may be influenced by the polarity of the solvent .
Result of Action
The molecular and cellular effects of 3HIQ’s action are primarily related to its role in excited state proton transfer mechanisms . This process can provide a possible explanation for the fluorescence quenching observed .
Action Environment
The action of 3HIQ is influenced by environmental factors such as the type of solvent used. For instance, in the presence of acetic acid, two protons of 3HIQ transfer along the dihydrogen bonds in the first excited state . This represents a different transfer mechanism to 3HIQ self-association .
Safety and Hazards
将来の方向性
特性
IUPAC Name |
2H-isoquinolin-3-one | |
---|---|---|
Source | PubChem | |
URL | https://pubchem.ncbi.nlm.nih.gov | |
Description | Data deposited in or computed by PubChem | |
InChI |
InChI=1S/C9H7NO/c11-9-5-7-3-1-2-4-8(7)6-10-9/h1-6H,(H,10,11) | |
Source | PubChem | |
URL | https://pubchem.ncbi.nlm.nih.gov | |
Description | Data deposited in or computed by PubChem | |
InChI Key |
GYPOFOQUZZUVQL-UHFFFAOYSA-N | |
Source | PubChem | |
URL | https://pubchem.ncbi.nlm.nih.gov | |
Description | Data deposited in or computed by PubChem | |
Canonical SMILES |
C1=CC2=CC(=O)NC=C2C=C1 | |
Source | PubChem | |
URL | https://pubchem.ncbi.nlm.nih.gov | |
Description | Data deposited in or computed by PubChem | |
Molecular Formula |
C9H7NO | |
Source | PubChem | |
URL | https://pubchem.ncbi.nlm.nih.gov | |
Description | Data deposited in or computed by PubChem | |
DSSTOX Substance ID |
DTXSID20997882 | |
Record name | Isoquinolin-3-ol | |
Source | EPA DSSTox | |
URL | https://comptox.epa.gov/dashboard/DTXSID20997882 | |
Description | DSSTox provides a high quality public chemistry resource for supporting improved predictive toxicology. | |
Molecular Weight |
145.16 g/mol | |
Source | PubChem | |
URL | https://pubchem.ncbi.nlm.nih.gov | |
Description | Data deposited in or computed by PubChem | |
Product Name |
3-Hydroxyisoquinoline | |
CAS RN |
7651-81-2 | |
Record name | 3(2H)-Isoquinolinone | |
Source | CAS Common Chemistry | |
URL | https://commonchemistry.cas.org/detail?cas_rn=7651-81-2 | |
Description | CAS Common Chemistry is an open community resource for accessing chemical information. Nearly 500,000 chemical substances from CAS REGISTRY cover areas of community interest, including common and frequently regulated chemicals, and those relevant to high school and undergraduate chemistry classes. This chemical information, curated by our expert scientists, is provided in alignment with our mission as a division of the American Chemical Society. | |
Explanation | The data from CAS Common Chemistry is provided under a CC-BY-NC 4.0 license, unless otherwise stated. | |
Record name | Isoquinolin-3-ol | |
Source | EPA DSSTox | |
URL | https://comptox.epa.gov/dashboard/DTXSID20997882 | |
Description | DSSTox provides a high quality public chemistry resource for supporting improved predictive toxicology. | |
Record name | 3-Hydroxyisoquinoline | |
Source | European Chemicals Agency (ECHA) | |
URL | https://echa.europa.eu/information-on-chemicals | |
Description | The European Chemicals Agency (ECHA) is an agency of the European Union which is the driving force among regulatory authorities in implementing the EU's groundbreaking chemicals legislation for the benefit of human health and the environment as well as for innovation and competitiveness. | |
Explanation | Use of the information, documents and data from the ECHA website is subject to the terms and conditions of this Legal Notice, and subject to other binding limitations provided for under applicable law, the information, documents and data made available on the ECHA website may be reproduced, distributed and/or used, totally or in part, for non-commercial purposes provided that ECHA is acknowledged as the source: "Source: European Chemicals Agency, http://echa.europa.eu/". Such acknowledgement must be included in each copy of the material. ECHA permits and encourages organisations and individuals to create links to the ECHA website under the following cumulative conditions: Links can only be made to webpages that provide a link to the Legal Notice page. | |
Synthesis routes and methods
Procedure details
Retrosynthesis Analysis
AI-Powered Synthesis Planning: Our tool employs the Template_relevance Pistachio, Template_relevance Bkms_metabolic, Template_relevance Pistachio_ringbreaker, Template_relevance Reaxys, Template_relevance Reaxys_biocatalysis model, leveraging a vast database of chemical reactions to predict feasible synthetic routes.
One-Step Synthesis Focus: Specifically designed for one-step synthesis, it provides concise and direct routes for your target compounds, streamlining the synthesis process.
Accurate Predictions: Utilizing the extensive PISTACHIO, BKMS_METABOLIC, PISTACHIO_RINGBREAKER, REAXYS, REAXYS_BIOCATALYSIS database, our tool offers high-accuracy predictions, reflecting the latest in chemical research and data.
Strategy Settings
Precursor scoring | Relevance Heuristic |
---|---|
Min. plausibility | 0.01 |
Model | Template_relevance |
Template Set | Pistachio/Bkms_metabolic/Pistachio_ringbreaker/Reaxys/Reaxys_biocatalysis |
Top-N result to add to graph | 6 |
Feasible Synthetic Routes
Q & A
Q1: What is the mechanism of phototautomerization in 3-hydroxyisoquinoline?
A3: Contrary to earlier suggestions that phototautomerization in 3-hydroxyisoquinoline occurs only in dimer or complex forms, recent studies reveal it can also happen in the excited state of the monomer via intramolecular proton transfer. [] This tautomerization is relatively slow, occurring on the nanosecond timescale. [] Furthermore, the process is influenced by solvent polarity and can occur in both the ground and excited states. []
Q2: How does complexation with water or acetic acid affect the tautomerization process of 3-hydroxyisoquinoline?
A4: Both water and acetic acid have a significant catalytic effect on the tautomerization of 3-hydroxyisoquinoline in its first excited singlet state. [] This proton transfer, a tunneling process, is strongly influenced by the movement of heavier atoms within the hydrogen bond bridges formed during complexation. [] Theoretical studies utilizing the multidimensional instanton model suggest that a multidimensional approach is necessary to accurately quantify this catalytic effect. []
Q3: Can you explain the double-proton transfer mechanism of 3-hydroxyisoquinoline in cyclohexane based on theoretical studies?
A5: Time-dependent density functional theory (TDDFT) calculations challenge the previously proposed double-proton transfer mechanism involving keto-enol complexes for 3-hydroxyisoquinoline self-association in cyclohexane. [] Instead, the theoretical results predict two forms of self-association: a normal enol/enol form and a tautomer keto/keto form. [] The high energy barrier between the enol and keto monomers in the ground state suggests the keto monomer should not exist in this state. [] Upon excitation, the enol/enol form undergoes a sequential proton transfer process, first in the excited state and then after transitioning to the ground state, leading to the more stable keto/keto form. []
Q4: How does the mechanism of proton transfer differ in 3-hydroxyisoquinoline when acetic acid is used as a solvent?
A6: Unlike the sequential transfer observed in cyclohexane, TDDFT studies show that in the presence of acetic acid, both protons of the 3-hydroxyisoquinoline/acetic acid complex transfer simultaneously along the dihydrogen bonds in the excited state. [] This distinct mechanism could explain the fluorescence quenching observed in acetic acid. []
Q5: How can 3-hydroxyisoquinoline be utilized in white light generation?
A7: 3-Hydroxyisoquinoline can act as an efficient donor in a Förster resonance energy transfer (FRET) system with Nile Red (NR) as the acceptor when embedded in a polyvinyl alcohol (PVA) matrix. [] When excited at 400 nm, the weak fluorescence of NR in PVA is significantly enhanced due to FRET from 3-hydroxyisoquinoline, while the emission of the donor is quenched. [] This energy transfer efficiency can be tuned by adjusting the relative concentrations of 3-hydroxyisoquinoline and Nile Red, enabling the creation of white light emission with specific CIE color coordinates. [] The resulting films demonstrate good photostability and mechanical flexibility, indicating potential for lighting applications. []
Q6: What is the molecular formula and weight of 3-hydroxyisoquinoline?
A6: The molecular formula of 3-hydroxyisoquinoline is C9H7NO, and its molecular weight is 145.16 g/mol.
Q7: What synthetic routes are available for preparing 3-hydroxyisoquinolines?
A7: Several synthetic approaches have been developed:
- Aryne Annulation: This method utilizes the reaction between functionalized enamines and arynes. The pathway can be controlled by the enamine's nitrogen substituent, leading to either indolines or isoquinolines. This strategy was successfully employed in the synthesis of papaverine, a pavine alkaloid, and the antitumor antibiotic (-)-quinocarcin. []
- One-Pot Aryne Acyl-Alkylation/Condensation: This approach utilizes β-ketoesters and arynes to produce 3-hydroxyisoquinolines and 2-hydroxy-1,4-naphthoquinones. The reaction proceeds through an aryne acyl-alkylation followed by an in situ condensation, offering a convenient two-step route from simple carboxylic acids. This method proved efficient in synthesizing the P,N-ligand QUINAP. [, ]
- Palladium-Catalyzed Enolate Arylation: This versatile method involves the coupling of an enolate with an ortho-functionalized aryl halide. The resulting intermediate, containing a protected 1,5-dicarbonyl moiety, can be cyclized to form various isoquinoline derivatives, including 3-hydroxyisoquinolines, depending on the nitrogen source used in the cyclization step. []
- Ugi Four-Component Reaction/Reductive Heck Cyclization: This strategy allows the preparation of diverse 3-hydroxyisoquinolines with blue fluorescence properties. It involves an initial Ugi multicomponent reaction followed by an intramolecular reductive Heck reaction, which can be carried out in a one-pot procedure. [, ]
Q8: What spectroscopic techniques are used to characterize 3-hydroxyisoquinoline?
A8: Various spectroscopic techniques have been employed to study 3-hydroxyisoquinoline:
- Ultraviolet Spectroscopy: This method was crucial in determining the tautomeric forms of 3-hydroxyisoquinoline in different solvents. []
- Infrared Spectroscopy (IR): IR spectroscopy, particularly in conjunction with matrix-isolation techniques, has been used to identify and distinguish between the tautomeric forms of 3-hydroxyisoquinoline. []
- Fluorescence Spectroscopy: This technique has been extensively used to investigate the excited-state properties of 3-hydroxyisoquinoline, including its phototautomerization behavior and its potential as a FRET donor in white light generation applications. [, ]
Q9: Have there been any studies on the biological activity of 3-hydroxyisoquinoline derivatives?
A9: Yes, some studies have investigated the biological activity of 3-hydroxyisoquinoline derivatives:
- Inhibition of HCV NS5b RNA-dependent RNA Polymerase: Research has focused on the potential of 3-hydroxyisoquinolines as inhibitors of the hepatitis C virus (HCV) NS5b RNA-dependent RNA polymerase, a key enzyme in viral replication. []
- Aza-2-aryl-1,4-naphthoquinone Derivatives as Arachidonic Acid Cascade Inhibitors: Studies have explored the ability of aza-2-aryl-1,4-naphthoquinone derivatives, structurally related to 3-hydroxyisoquinoline, to inhibit enzymes involved in the arachidonic acid cascade, such as 5-lipoxygenase (5-LO), 12-lipoxygenase (12-LO), cyclooxygenase-1 (COX-1), and cytosolic phospholipase A2 (cPLA2). These studies aim to develop selective inhibitors for treating inflammatory diseases. [, ]
- Fluorescence Properties in Biological Systems: A novel highly functionalized 3-hydroxyisoquinoline derivative was synthesized and its fluorescence properties were investigated in the ascidian Ciona intestinalis. This compound exhibited blue fluorescence and localized in the cell nuclei of larval tissues, highlighting its potential as a fluorescent dye for biological imaging. []
試験管内研究製品の免責事項と情報
BenchChemで提示されるすべての記事および製品情報は、情報提供を目的としています。BenchChemで購入可能な製品は、生体外研究のために特別に設計されています。生体外研究は、ラテン語の "in glass" に由来し、生物体の外で行われる実験を指します。これらの製品は医薬品または薬として分類されておらず、FDAから任何の医療状態、病気、または疾患の予防、治療、または治癒のために承認されていません。これらの製品を人間または動物に体内に導入する形態は、法律により厳格に禁止されています。これらのガイドラインに従うことは、研究と実験において法的および倫理的な基準の遵守を確実にするために重要です。