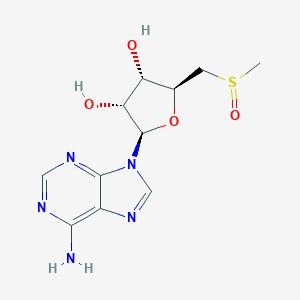
Methylthioadenosine sulfoxide
概要
説明
Methylthioadenosine sulfoxide (MTA-SO) is a sulfur-containing nucleoside derivative identified as 5'-deoxy-5'-methylthioadenosine sulfoxide (Fig. 1) . It is structurally related to 5'-methylthioadenosine (MTA), a byproduct of polyamine biosynthesis derived from S-adenosylmethionine (SAM) . The sulfoxide group in MTA-SO arises from the oxidation of the methylthio (-S-CH₃) moiety in MTA, forming a sulfoxide (-SO-CH₃). This modification alters its chemical reactivity and biological interactions. MTA-SO is detected in metabolic profiling studies, particularly in cancer cell lines like MCF-7, where it co-occurs with MTA, SAM, and S-adenosylhomocysteine (SAH) . Its role is hypothesized to intersect with oxidative stress regulation and methionine salvage pathways, though mechanistic details remain under investigation.
準備方法
Chemical Oxidation Methods
Hydrogen Peroxide with Acetic Acid
The oxidation of MTA to MTA-SO using hydrogen peroxide (H₂O₂) in glacial acetic acid is a widely documented method. This approach leverages the electrophilic nature of protonated hydrogen peroxide to selectively oxidize the sulfide group without over-oxidation to sulfone. Key parameters include:
-
Reagents : 30–50% aqueous H₂O₂, glacial acetic acid.
-
Conditions : Room temperature, 1–2 hours.
A study by Mazandaran University demonstrated that this method avoids sulfone formation due to the stability of sulfoxides under acidic conditions. The absence of metal catalysts simplifies purification, making it suitable for laboratory-scale synthesis .
Catalytic Oxidation with LiNbMoO₆
A patented method employs lithium niobium molybdate (LiNbMoO₆) as a catalyst with H₂O₂ in methanol. This system is particularly effective for allylic sulfides but has been adapted for nucleoside derivatives like MTA:
-
Catalyst Loading : 5 mol% LiNbMoO₆.
-
Conditions : 0°C, 1–4 hours.
-
Yield : 85–92% with 94–99% enantiomeric excess (ee) for chiral sulfoxides .
This method’s selectivity stems from the catalyst’s ability to stabilize transition states, preventing epoxidation of adjacent double bonds in complex substrates .
Biocatalytic Synthesis
Methionine Sulfoxide Reductase A (MsrA)
Recombinant Pseudomonas monteilii MsrA enables asymmetric reductive resolution of racemic sulfoxides. This enzymatic method is notable for its sustainability and high substrate tolerance:
-
Substrate Concentration : Up to 200 mM (≈32 g/L).
-
Conditions : pH 7.0, 30°C, 4 hours.
-
Yield : 43–46% (theoretical maximum 50%) with 94–99% ee for (R)-sulfoxides .
The enzyme regeneration system using dithiothreitol (DTT) enhances scalability, addressing limitations in traditional biocatalytic oxidation .
One-Pot Synthesis Approaches
Sulfinyl-Dication Intermediate Strategy
A one-pot method utilizing sulfur dioxide (DABSO) and organometallic reagents generates sulfoxides via a sulfinate ester intermediate. Adapted for MTA-SO:
-
Reagents : DABSO, TMS-Cl, Grignard reagents.
-
Conditions : Room temperature, 18–25 hours.
-
Yield : 80–85% .
This method avoids thiol intermediates, reducing byproduct formation and streamlining purification .
Industrial-Scale Production Considerations
Continuous Flow Reactors
Industrial protocols emphasize reactor design to manage exothermic reactions and improve yield:
-
Catalytic Distillation : Titanium silicon molecular sieves in a multi-zone reactor enhance dimethyl sulfide oxidation efficiency, achieving 95% conversion .
-
Temperature Control : Maintaining ≤120°C prevents decomposition of heat-sensitive intermediates .
Solvent and Catalyst Recycling
Ethanol-water mixtures and reusable catalysts (e.g., LiNbMoO₆) reduce waste. A green metrics analysis reported an E-factor of 9.6 and PMI of 10.6 for scaled sulfoxide synthesis .
Comparative Analysis of Methods
Method | Yield (%) | Selectivity | Scalability | Cost Efficiency |
---|---|---|---|---|
H₂O₂/Acetic Acid | 90–96 | >99% | Moderate | High |
LiNbMoO₆/H₂O₂ | 85–92 | 94–99% ee | High | Moderate |
Biocatalytic (MsrA) | 43–46 | 94–99% ee | High | Low |
One-Pot (DABSO) | 80–85 | 95% | Low | Moderate |
Key Findings :
科学的研究の応用
Cancer Research
MTA has been extensively studied for its role in cancer biology, particularly in relation to polyamine metabolism. Polyamines are critical for cell growth and differentiation, and MTA acts as an inhibitor of polyamine biosynthesis.
Case Study: Chemoprotective Agent
A recent study highlighted the potential of MTA as a chemoprotective agent against the toxic side effects of fluorouracil (5-FU) in MTAP-deficient cancers. MTA was shown to selectively protect against 5-FU-induced anemia without interfering with the drug's efficacy on cancer cells, demonstrating its potential in combination therapies for cancer treatment .
Study | Findings | Implications |
---|---|---|
Zhang et al. (2024) | MTA protects against 5-FU toxicity | Supports use in MTAP-deficient cancers |
Neuroprotection
MTA has demonstrated neuroprotective effects in various experimental models. It is produced during the metabolism of S-adenosylmethionine and has been shown to cross the blood-brain barrier.
Case Study: Neuroinflammation
In models of experimental autoimmune encephalomyelitis (EAE), MTA exhibited significant immunomodulatory activity, outperforming some approved therapies for multiple sclerosis. This suggests that MTA may be beneficial in treating neuroinflammatory conditions .
Study | Findings | Implications |
---|---|---|
PLOS One (2014) | MTA effective in EAE models | Potential therapeutic for multiple sclerosis |
Immune Regulation
MTA has been implicated in immune responses, particularly regarding T cell function. It suppresses T cell activity, which can be detrimental in cancer but beneficial in autoimmune diseases.
Case Study: T Cell Function
Research indicated that systemic administration of enzymes that deplete MTA could restore T cell function, suggesting a therapeutic angle for enhancing immune responses in cancer therapy .
Study | Findings | Implications |
---|---|---|
PMC (2018) | Depletion of MTA restores T cell activity | Enhances potential for cancer immunotherapy |
Microbial Pathogenesis
MTA plays a dual role in host-pathogen interactions, particularly with Salmonella Typhimurium. It has been shown to regulate bacterial virulence through its effects on methionine metabolism.
Case Study: Salmonella Virulence
Studies demonstrated that high levels of MTA could suppress Salmonella virulence by inhibiting its motility and invasion capabilities during infection. This highlights MTA's potential as a therapeutic target for managing bacterial infections .
Study | Findings | Implications |
---|---|---|
PMC (2018) | MTA reduces Salmonella virulence | Potential target for infection control |
Other Biological Activities
Beyond these primary applications, MTA has shown promise in other areas such as:
作用機序
Methylthioadenosine sulfoxide exerts its effects through various molecular targets and pathways:
Anti-inflammatory: It modulates the activity of enzymes involved in the inflammatory response, reducing the production of pro-inflammatory cytokines.
Anti-cancer: It inhibits key signaling pathways that promote tumor cell growth and survival, such as the Akt and MAPK pathways. It also induces apoptosis by activating caspases and other pro-apoptotic proteins.
類似化合物との比較
Structural and Functional Comparison with Key Analogues
MTA-SO vs. 5'-Methylthioadenosine (MTA)
- Metabolic Pathways: MTA is a key intermediate in the methionine salvage pathway, recycled via methylthioadenosine phosphorylase (MTAP) to adenine and methylthioribose-1-phosphate .
- Biological Activity : MTA exhibits antiproliferative effects in leukemia cells (e.g., inhibition of dimethyl sulfoxide-induced differentiation in Friend erythroleukemic cells at IC₅₀ ~10 μM) . MTA-SO’s activity is less characterized but may modulate redox signaling due to its sulfoxide group .
MTA-SO vs. Methionine Sulfoxide
- Oxidative Stress Connection : Methionine sulfoxide is a well-established biomarker of oxidative damage, reversibly reduced by Msr enzymes . MTA-SO’s detection in metabolic stress conditions (e.g., cancer cells) suggests a parallel role, but its reduction pathway remains unclear .
MTA-SO vs. SAM and SAH
- Metabolic Network : SAM donates methyl groups in polyamine synthesis, generating MTA as a byproduct. MTA-SO may represent a terminal oxidation product, diverting MTA from salvage pathways under oxidative conditions .
Research Findings and Implications
MTA-SO in Disease Models
- Cancer Metabolism : MTA-SO is elevated in MCF-7 breast cancer cells alongside MTA and SAM, suggesting its role in dysregulated nucleoside metabolism .
- Antioxidant vs. Pro-Oxidant Effects : Similar to methionine sulfoxide, MTA-SO may exhibit dual roles depending on cellular redox status, though this requires validation .
Enzymatic Interactions
- MTA Metabolism : MTA is processed by MTAP in salvage pathways , while MTA-SO’s fate may involve sulfoxide-specific reductases or oxidases yet to be identified.
- Comparative Inhibition : MTA inhibits erythroleukemic cell differentiation at low concentrations (10–400 μM) , whereas MTA-SO’s effects are undefined but structurally distinct due to the sulfoxide group.
Data Tables
Table 1: Structural and Functional Comparison of Sulfur-Containing Metabolites
Table 2: Enzymatic Activities on Key Compounds
生物活性
Methylthioadenosine sulfoxide (MTA sulfoxide) is a compound derived from the metabolism of S-adenosylmethionine, playing a significant role in various biological processes. This article explores the biological activity of MTA sulfoxide, focusing on its mechanisms of action, therapeutic potential, and implications in disease models.
Overview of this compound
This compound is a sulfur-containing nucleoside that is involved in the polyamine metabolism pathway. It is produced from 5'-deoxy-5'-methylthioadenosine (MTA) and is known for its diverse biological effects, particularly in the context of immunomodulation and neuroprotection.
- Polyamine Metabolism Inhibition : MTA sulfoxide acts as an inhibitor of polyamine biosynthesis, which is crucial for cell growth and differentiation. This inhibition can lead to altered cellular functions, particularly in cancer cells where polyamines are often overproduced.
- Neuroprotective Effects : Studies have shown that MTA sulfoxide can cross the blood-brain barrier and exert neuroprotective effects in models of neurodegenerative diseases. It has been demonstrated to reduce excitotoxicity in neuronal cultures and protect against hypoxic brain damage in vivo .
- Immunomodulatory Properties : MTA sulfoxide has significant immunomodulatory effects, particularly on natural killer (NK) cells. It inhibits NK cell cytotoxicity and cytokine production by interfering with signaling pathways such as PI3K/AKT and MAPK/ERK, which are vital for NK cell activation .
Neurodegenerative Diseases
Research indicates that MTA sulfoxide has potential therapeutic effects in neurodegenerative diseases such as Parkinson's disease and multiple sclerosis. In experimental models, it has been shown to improve neurological outcomes by reducing inflammation and promoting neuronal survival .
Cancer
In cancer research, the deficiency of methylthioadenosine phosphorylase (MTAP), which metabolizes MTA, creates a metabolic vulnerability that can be exploited therapeutically. Tumors lacking MTAP show increased sensitivity to antifolate therapies like pemetrexed, suggesting that targeting MTA metabolism could enhance cancer treatment efficacy .
Study | Findings | Implications |
---|---|---|
PLOS One Study (2014) | MTA shows neuroprotective effects against excitotoxicity | Potential treatment for neurodegenerative diseases |
Nature Communications (2022) | MTAP deficiency correlates with improved response to pemetrexed | Targeting MTA metabolism in cancer therapy |
Frontiers in Immunology (2020) | MTA inhibits NK cell functions | Implications for cancer immunotherapy |
Case Studies
- Neuroprotection in Parkinson's Disease : In animal models, administration of MTA sulfoxide resulted in reduced dopaminergic neuron loss and improved motor function, indicating its potential as a protective agent against neurodegeneration .
- Cancer Treatment Synergy : A study involving MTAP-deficient lung adenocarcinoma cells demonstrated that these cells exhibited higher apoptosis rates when treated with pemetrexed compared to MTAP-proficient cells, highlighting the potential for combination therapies that include MTA sulfoxide .
- Infection Response Modulation : In Salmonella infection models, elevated levels of extracellular MTA were linked to both pro-inflammatory responses and protective effects against sepsis, illustrating the dual role of MTA sulfoxide in host-pathogen interactions .
化学反応の分析
Oxidation Reactions
Methylthioadenosine sulfoxide can undergo various oxidation reactions, which are vital for its transformation into other biologically active compounds. The oxidation of methylthioadenosine to its sulfoxide form typically involves the following pathways:
-
Direct Oxidation : The oxidation process can be facilitated by oxidizing agents such as hydrogen peroxide or dimethyl sulfoxide (DMSO). In aqueous solutions, the sulfur radical cations produced during photochemical reactions can lead to sulfoxide formation through interactions with superoxide radicals .
-
Biocatalytic Oxidation : Enzymes such as sulfoxide reductases play a critical role in the biological oxidation of methylthioadenosine. These enzymes can facilitate the conversion of methylthioadenosine to its sulfoxide form under mild conditions, highlighting the importance of enzymatic activity in metabolic pathways .
Reduction Reactions
This compound can also undergo reduction back to methylthioadenosine, primarily through enzymatic pathways:
-
Methionine Sulfoxide Reductase : This enzyme catalyzes the reduction of sulfoxides back to their corresponding sulfides, which is essential for maintaining cellular redox balance and preventing oxidative damage . The reaction can be summarized as follows:
Substitution Reactions
This compound can participate in nucleophilic substitution reactions, particularly when exposed to nucleophiles in the presence of Lewis acids:
-
Electrophilic Substitution : The presence of an aromatic system adjacent to the activated sulfoxide allows nucleophiles to attack the aromatic ring, leading to the formation of substituted aryl sulfides. This reaction pathway is essential for synthesizing complex organic molecules .
Pummerer Rearrangement
One notable reaction involving this compound is the Pummerer rearrangement, where it can convert into aldehydes under acidic conditions:
-
Mechanism : In this rearrangement, this compound reacts with acids to form a sulfonium ion intermediate, which subsequently rearranges to yield aldehyde products .
Data Tables of Reaction Pathways
Reaction Type | Reactants | Products | Conditions |
---|---|---|---|
Oxidation | Methylthioadenosine | This compound | H₂O₂ or DMSO |
Reduction | This compound | Methylthioadenosine | Catalyzed by Msr |
Substitution | This compound + Nucleophile | Aryl Sulfides | Lewis Acid |
Pummerer Rearrangement | This compound | Aldehydes | Acidic conditions |
Research Findings and Implications
Recent studies have highlighted the significance of this compound in various biochemical pathways, particularly in relation to its role in redox biology and sulfur metabolism. The ability of this compound to undergo multiple types of reactions makes it a valuable intermediate in synthetic organic chemistry and pharmaceuticals.
-
Biological Impact : The oxidation and reduction processes involving this compound are crucial for maintaining cellular health and function, particularly in relation to oxidative stress responses.
-
Synthetic Applications : Understanding these chemical reactions opens avenues for developing new synthetic strategies for creating complex molecules with potential therapeutic applications.
Q & A
Q. What methodological approaches are recommended for quantifying Methylthioadenosine sulfoxide (MTA sulfoxide) in biological samples, given its oxidative instability?
Basic Research Focus
To mitigate oxidation during quantification, researchers should employ liquid chromatography-tandem mass spectrometry (LC-MS/MS) with electrospray ionization (ESI) in negative ion mode. Sample preparation must occur under inert conditions (e.g., nitrogen atmosphere), supplemented with antioxidants like dithiothreitol (DTT) or ethylenediaminetetraacetic acid (EDTA) to stabilize MTA sulfoxide. Internal standards, such as isotopically labeled MTA sulfoxide (e.g., ¹³C or ¹⁵N analogs), improve accuracy. Pre-analytical steps should include rapid freezing of tissues and acidification to pH 3–4 to inhibit enzymatic degradation .
Q. How can researchers reconcile contradictory findings on MTA sulfoxide’s role as an oxidative stress biomarker across model organisms?
Advanced Research Focus
Discrepancies may arise from species-specific metabolic flux variations or differences in experimental stress induction (e.g., chemical vs. genetic oxidative stress models). To address this, comparative metabolomic studies should standardize stress conditions (e.g., hydrogen peroxide concentration, exposure duration) and incorporate orthogonal validation methods, such as genetic knockouts of methionine sulfoxide reductase (MSR) or siRNA silencing. Cross-species pathway analysis using tools like KEGG or MetaCyc can identify conserved metabolic interactions, while multivariate statistics (e.g., PCA or OPLS-DA) highlight context-dependent regulatory nodes .
Q. What experimental designs are optimal for mapping MTA sulfoxide’s interconversion with methionine and S-adenosylmethionine (SAM)?
Advanced Research Focus
Stable isotope tracing (e.g., ¹³C-methionine or ³⁴S-labeled SAM) coupled with time-course metabolomics can track dynamic flux in the methionine salvage pathway. For in vitro systems, enzymatic assays using purified MTA phosphorylase or SAM synthetase under controlled redox conditions (e.g., glutathione redox buffers) clarify kinetic parameters. In vivo, CRISPR/Cas9-generated Arabidopsis or Drosophila mutants lacking key salvage enzymes (e.g., MTAP) can isolate MTA sulfoxide’s metabolic contributions. Data integration via metabolic network modeling (e.g., COBRA Toolbox) enhances pathway resolution .
Q. How does pH and temperature variability during sample processing affect MTA sulfoxide measurement accuracy, and what stabilization strategies are validated?
Basic Research Focus
MTA sulfoxide degrades rapidly above 4°C and at neutral-to-alkaline pH. Immediate snap-freezing in liquid nitrogen and storage at -80°C are critical. Acidic extraction buffers (e.g., 0.1% formic acid in methanol) minimize oxidation. Additives like sodium ascorbate (1 mM) or catalase inhibitors (e.g., 3-amino-1,2,4-triazole) improve recovery rates. Method validation should include spike-and-recovery experiments in biological matrices (e.g., plasma, plant homogenates) to assess intra-assay variability .
Q. What advanced statistical frameworks are suitable for correlating MTA sulfoxide levels with oxidative stress biomarkers in multi-omics datasets?
Advanced Research Focus
Partial least squares-discriminant analysis (PLS-DA) and weighted gene co-expression network analysis (WGCNA) can identify covarying metabolites and genes in transcriptome-metabolome datasets. For longitudinal studies, mixed-effects models account for intra-subject variability. Pathway enrichment tools (e.g., MetaboAnalyst 5.0) contextualize MTA sulfoxide within oxidative stress pathways, while machine learning approaches (e.g., random forest) prioritize biomarkers with predictive power for redox imbalance. Reproducibility requires adherence to FAIR data principles and cross-validation against independent cohorts .
Q. How can in vitro and in vivo models be optimized to study MTA sulfoxide’s dual role in redox regulation and polyamine synthesis?
Advanced Research Focus
In vitro, HepG2 or HEK293 cells treated with polyamine synthesis inhibitors (e.g., DFMO) can dissect MTA sulfoxide’s feedback mechanisms. For in vivo models, Drosophila TRPA1 mutants (impaired redox sensing) or Arabidopsis atleuc1-1 mutants (blocked methionine salvage) provide genetic context. Dual RNAi knockdowns targeting both MTA sulfoxide reductases and polyamine transporters clarify crosstalk. Real-time redox imaging (e.g., roGFP2-Orp1 probes) paired with LC-MS quantitation resolves spatiotemporal dynamics .
特性
IUPAC Name |
(2R,3R,4S,5S)-2-(6-aminopurin-9-yl)-5-(methylsulfinylmethyl)oxolane-3,4-diol | |
---|---|---|
Source | PubChem | |
URL | https://pubchem.ncbi.nlm.nih.gov | |
Description | Data deposited in or computed by PubChem | |
InChI |
InChI=1S/C11H15N5O4S/c1-21(19)2-5-7(17)8(18)11(20-5)16-4-15-6-9(12)13-3-14-10(6)16/h3-5,7-8,11,17-18H,2H2,1H3,(H2,12,13,14)/t5-,7-,8-,11-,21?/m1/s1 | |
Source | PubChem | |
URL | https://pubchem.ncbi.nlm.nih.gov | |
Description | Data deposited in or computed by PubChem | |
InChI Key |
WXOJULRVRHWMGT-JLQSGANNSA-N | |
Source | PubChem | |
URL | https://pubchem.ncbi.nlm.nih.gov | |
Description | Data deposited in or computed by PubChem | |
Canonical SMILES |
CS(=O)CC1C(C(C(O1)N2C=NC3=C(N=CN=C32)N)O)O | |
Source | PubChem | |
URL | https://pubchem.ncbi.nlm.nih.gov | |
Description | Data deposited in or computed by PubChem | |
Isomeric SMILES |
CS(=O)C[C@@H]1[C@H]([C@H]([C@@H](O1)N2C=NC3=C(N=CN=C32)N)O)O | |
Source | PubChem | |
URL | https://pubchem.ncbi.nlm.nih.gov | |
Description | Data deposited in or computed by PubChem | |
Molecular Formula |
C11H15N5O4S | |
Source | PubChem | |
URL | https://pubchem.ncbi.nlm.nih.gov | |
Description | Data deposited in or computed by PubChem | |
DSSTOX Substance ID |
DTXSID50955455 | |
Record name | 9-[5-Deoxy-5-(methanesulfinyl)pentofuranosyl]-9H-purin-6-amine | |
Source | EPA DSSTox | |
URL | https://comptox.epa.gov/dashboard/DTXSID50955455 | |
Description | DSSTox provides a high quality public chemistry resource for supporting improved predictive toxicology. | |
Molecular Weight |
313.34 g/mol | |
Source | PubChem | |
URL | https://pubchem.ncbi.nlm.nih.gov | |
Description | Data deposited in or computed by PubChem | |
CAS No. |
3387-65-3 | |
Record name | 5′-Deoxy-5′-(methylsulfinyl)adenosine | |
Source | CAS Common Chemistry | |
URL | https://commonchemistry.cas.org/detail?cas_rn=3387-65-3 | |
Description | CAS Common Chemistry is an open community resource for accessing chemical information. Nearly 500,000 chemical substances from CAS REGISTRY cover areas of community interest, including common and frequently regulated chemicals, and those relevant to high school and undergraduate chemistry classes. This chemical information, curated by our expert scientists, is provided in alignment with our mission as a division of the American Chemical Society. | |
Explanation | The data from CAS Common Chemistry is provided under a CC-BY-NC 4.0 license, unless otherwise stated. | |
Record name | Methylthioadenosine sulfoxide | |
Source | ChemIDplus | |
URL | https://pubchem.ncbi.nlm.nih.gov/substance/?source=chemidplus&sourceid=0003387653 | |
Description | ChemIDplus is a free, web search system that provides access to the structure and nomenclature authority files used for the identification of chemical substances cited in National Library of Medicine (NLM) databases, including the TOXNET system. | |
Record name | 9-[5-Deoxy-5-(methanesulfinyl)pentofuranosyl]-9H-purin-6-amine | |
Source | EPA DSSTox | |
URL | https://comptox.epa.gov/dashboard/DTXSID50955455 | |
Description | DSSTox provides a high quality public chemistry resource for supporting improved predictive toxicology. | |
Retrosynthesis Analysis
AI-Powered Synthesis Planning: Our tool employs the Template_relevance Pistachio, Template_relevance Bkms_metabolic, Template_relevance Pistachio_ringbreaker, Template_relevance Reaxys, Template_relevance Reaxys_biocatalysis model, leveraging a vast database of chemical reactions to predict feasible synthetic routes.
One-Step Synthesis Focus: Specifically designed for one-step synthesis, it provides concise and direct routes for your target compounds, streamlining the synthesis process.
Accurate Predictions: Utilizing the extensive PISTACHIO, BKMS_METABOLIC, PISTACHIO_RINGBREAKER, REAXYS, REAXYS_BIOCATALYSIS database, our tool offers high-accuracy predictions, reflecting the latest in chemical research and data.
Strategy Settings
Precursor scoring | Relevance Heuristic |
---|---|
Min. plausibility | 0.01 |
Model | Template_relevance |
Template Set | Pistachio/Bkms_metabolic/Pistachio_ringbreaker/Reaxys/Reaxys_biocatalysis |
Top-N result to add to graph | 6 |
Feasible Synthetic Routes
試験管内研究製品の免責事項と情報
BenchChemで提示されるすべての記事および製品情報は、情報提供を目的としています。BenchChemで購入可能な製品は、生体外研究のために特別に設計されています。生体外研究は、ラテン語の "in glass" に由来し、生物体の外で行われる実験を指します。これらの製品は医薬品または薬として分類されておらず、FDAから任何の医療状態、病気、または疾患の予防、治療、または治癒のために承認されていません。これらの製品を人間または動物に体内に導入する形態は、法律により厳格に禁止されています。これらのガイドラインに従うことは、研究と実験において法的および倫理的な基準の遵守を確実にするために重要です。