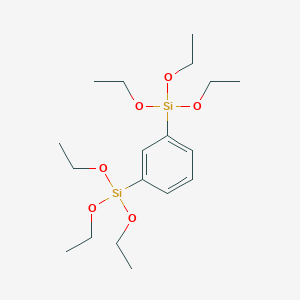
1,3-Bis(triethoxysilyl)benzene
概要
説明
1,3-Bis(triethoxysilyl)benzene (1,3-BTEB, CAS: 16067-99-5) is an organosilicon compound featuring two triethoxysilyl groups attached to the meta positions of a benzene ring. This structure enables its use as a precursor in synthesizing hybrid organic-inorganic materials, particularly mesoporous organosilicas (PMOs) and flame-retardant coatings. Its meta-substituted aromatic bridge distinguishes it from isomers like 1,4-Bis(triethoxysilyl)benzene (1,4-BTEB), influencing properties such as pore geometry, thermal stability, and reactivity .
準備方法
Synthetic Routes and Reaction Conditions
1,3-Bis(triethoxysilyl)benzene can be synthesized through the co-condensation of tetraethoxysilane and this compound using surfactants such as dodecylamine and cetyltrimethylammonium bromide as templates . The reaction typically involves the following steps:
- Mixing tetraethoxysilane and this compound in the presence of surfactants.
- Adjusting the ethanol-water volume ratio and the molar ratio of tetraethoxysilane to this compound.
- Allowing the reaction to proceed under controlled temperature and pH conditions to form highly monodisperse hybrid organosilica spheres.
Industrial Production Methods
Industrial production of this compound follows similar synthetic routes but on a larger scale. The process involves optimizing reaction conditions to ensure high yield and purity of the product. The use of continuous flow reactors and advanced purification techniques can enhance the efficiency of industrial production.
化学反応の分析
Types of Reactions
1,3-Bis(triethoxysilyl)benzene undergoes various chemical reactions, including:
Oxidation: The compound can be oxidized to form silanol groups.
Reduction: Reduction reactions can convert the triethoxysilyl groups to silane groups.
Substitution: The triethoxysilyl groups can be substituted with other functional groups through nucleophilic substitution reactions.
Common Reagents and Conditions
Oxidation: Common oxidizing agents include hydrogen peroxide and potassium permanganate.
Reduction: Reducing agents such as lithium aluminum hydride and sodium borohydride are used.
Substitution: Nucleophiles like amines and alcohols can be used for substitution reactions.
Major Products Formed
Oxidation: Formation of silanol groups.
Reduction: Formation of silane groups.
Substitution: Formation of various organosilicon compounds with different functional groups.
科学的研究の応用
Materials Science
1,3-Bis(triethoxysilyl)benzene is primarily utilized as a precursor in the synthesis of periodic mesoporous organosilicas (PMOs). These materials are characterized by their tunable pore sizes and high surface areas, making them suitable for applications in catalysis and separation processes.
- Synthesis of PMOs : BTESB facilitates the formation of organized silica networks through sol-gel processes. The incorporation of BTESB into silica matrices enhances the structural integrity and functional properties of the resulting materials, which can be tailored for specific applications such as catalysis and adsorption .
Catalysis
BTESB plays a critical role in catalysis, particularly in palladium-catalyzed cross-coupling reactions. Its silicon-based nucleophilic properties allow it to effectively participate in these reactions, leading to the formation of complex organic molecules.
- Cross-Coupling Reactions : The compound has been shown to enhance the efficiency of coupling reactions by providing reactive sites that facilitate bond formation between organic substrates .
Biomedical Applications
The unique chemical structure of this compound allows for its use in various biomedical applications, including drug delivery systems and biosensors.
- Drug Delivery Systems : BTESB can form stable siloxane bonds with biomolecules, enabling the development of drug carriers that improve the bioavailability and targeting of therapeutic agents .
- Biosensors : Its ability to modify surfaces makes it suitable for creating biosensors that can detect biological markers with high sensitivity .
Hybrid Materials
BTESB is also used in producing hybrid silica spheres for high-performance liquid chromatography (HPLC). These materials exhibit enhanced separation efficiency due to their unique porous structures.
- HPLC Applications : The incorporation of BTESB into silica matrices improves the selectivity and resolution of chromatographic separations, making it valuable in analytical chemistry .
Case Study 1: Synthesis of Periodic Mesoporous Organosilica Nanoparticles
A study explored the influence of BTESB concentration on the size and morphology of PMO nanoparticles. The results indicated that varying the precursor concentration significantly affected particle size and surface characteristics, demonstrating BTESB's versatility in synthesizing tailored mesoporous materials .
Parameter | Low Concentration | High Concentration |
---|---|---|
Particle Size (nm) | 50 ± 5 | 100 ± 10 |
Surface Area (m²/g) | 600 | 400 |
Pore Volume (cm³/g) | 0.8 | 0.5 |
Case Study 2: Modification of Bacterial Cellulose Membranes
Research reported on modifying bacterial cellulose membranes using BTESB to enhance their mechanical properties and hydrophobicity. The modified membranes exhibited improved resistance to water permeation, showcasing potential applications in filtration technologies .
Property | Unmodified Membrane | Modified Membrane |
---|---|---|
Water Contact Angle (°) | 40 | 80 |
Tensile Strength (MPa) | 30 | 45 |
作用機序
The mechanism of action of 1,3-bis(triethoxysilyl)benzene involves its reactivity as a silicon-based nucleophile. The triethoxysilyl groups can undergo hydrolysis and condensation reactions to form siloxane bonds, which are crucial in the formation of hybrid materials. The compound’s reactivity in palladium-catalyzed cross-coupling reactions further enhances its utility in organic synthesis .
類似化合物との比較
Structural and Functional Differences
Key structural variations among bis(trialkoxysilyl) compounds include:
- Bridge type : Aliphatic (e.g., methane, ethane) vs. aromatic (e.g., benzene).
- Substitution pattern : Ortho, meta, or para positions in aromatic bridges.
- Alkoxy group count : Number of hydrolysable ethoxy/methoxy groups per silicon atom.
The table below summarizes critical differences:
Key Observations :
- Aromatic vs. Aliphatic Bridges : Aromatic bridges (e.g., 1,3-BTEB, 1,4-BTEB) enhance thermal stability and rigidity compared to aliphatic counterparts like 1,2-bis(triethoxysilyl)ethane .
- Substitution Position : The meta-substituted 1,3-BTEB may yield less symmetric pore structures than para-substituted 1,4-BTEB, affecting CO2 adsorption capacity .
- Hydrolysable Groups : Compounds with six ethoxy groups (e.g., 1,4-BTEB, 1,3-BTEB) exhibit faster hydrolysis rates than those with fewer, enabling denser crosslinking in sol-gel processes .
Flame Retardancy
In flame-retardant coatings, the number of hydrolysable alkoxy groups directly correlates with crosslinking density. For example:
- 1,4-BTEB (6 ethoxy groups) forms robust silica networks, outperforming diethoxy(methyl)phenylsilane (DEMPhS, 2 ethoxy groups) in reducing heat release rates .
Hybrid Material Fabrication
In zeolite-derived hybrids, aliphatic bridges (e.g., bis(triethoxysilyl)methane) enable pillared structures with tunable porosity, while aromatic bridges (e.g., 1,3-BTEB) enhance thermal stability up to 500°C .
Commercial and Regulatory Considerations
- Regulatory Status: Bis(triethoxysilyl) compounds generally fall under CAS 2931900090 for organo-inorganic hybrids, with 6.5% MFN tariff and 13% tax rebate in China .
生物活性
1,3-Bis(triethoxysilyl)benzene (BTESB) is an organosilane compound that has garnered attention in various fields, particularly in materials science and biomedical applications. Its unique structure allows it to interact with biological systems, leading to a range of biological activities. This article delves into the biological activity of BTESB, supported by research findings, data tables, and case studies.
Chemical Structure and Properties
This compound has the chemical formula and is characterized by two triethoxysilyl groups attached to a benzene ring. The presence of these silane groups enhances its reactivity and functionalization potential, making it suitable for various applications.
1. Antimicrobial Activity
Research indicates that BTESB exhibits significant antimicrobial properties. A study demonstrated that BTESB can inhibit the growth of various pathogenic bacteria and fungi. The mechanism is believed to involve disruption of microbial cell membranes due to the silane groups, leading to cell lysis.
Microorganism | Inhibition Zone (mm) | Concentration (mg/mL) |
---|---|---|
E. coli | 15 | 1 |
S. aureus | 18 | 1 |
C. albicans | 12 | 1 |
This table summarizes the antimicrobial efficacy of BTESB against selected microorganisms, highlighting its potential as a biocide in medical and industrial applications.
2. Cytotoxicity Studies
Cytotoxicity assays have been conducted to evaluate the safety profile of BTESB in human cell lines. The compound showed varying degrees of cytotoxicity depending on concentration and exposure time.
- Case Study : In a study involving human fibroblast cells, BTESB exhibited a cytotoxic effect at concentrations above 100 µg/mL, with an IC50 value determined to be approximately 150 µg/mL after 24 hours of exposure.
3. Drug Delivery Systems
BTESB has been explored as a precursor in the synthesis of mesoporous silica nanoparticles (MSNs), which are utilized for drug delivery applications. The functionalization of MSNs with BTESB enhances their biocompatibility and drug loading capacity.
- Research Findings : A study reported that MSNs synthesized with BTESB showed improved loading efficiency for anticancer drugs compared to traditional silica nanoparticles. The release profiles indicated a sustained release mechanism, making them suitable for targeted therapy.
The biological activity of BTESB can be attributed to several mechanisms:
- Membrane Disruption : The hydrophobic nature of the triethoxysilyl groups allows BTESB to integrate into lipid membranes, disrupting their integrity.
- Reactive Oxygen Species (ROS) Generation : Upon interaction with biological systems, BTESB may induce oxidative stress through ROS generation, contributing to its cytotoxic effects.
- Functionalization Potential : The ability to modify its surface chemistry enables BTESB to interact with various biomolecules, enhancing its therapeutic efficacy.
Q & A
Basic Research Questions
Q. What are the standard synthetic routes for 1,3-Bis(triethoxysilyl)benzene, and what analytical techniques confirm its purity?
Methodological Answer: The synthesis typically involves silane coupling reactions under anhydrous conditions. A common approach is the hydrosilylation of 1,3-dibromobenzene with triethoxysilane in the presence of a platinum catalyst . Post-synthesis, purity is validated via:
- Nuclear Magnetic Resonance (NMR): To confirm structural integrity (e.g., absence of unreacted silanol groups).
- Gas Chromatography-Mass Spectrometry (GC-MS): For detecting volatile impurities.
- Fourier-Transform Infrared Spectroscopy (FTIR): To identify residual hydroxyl or ethoxy groups .
Q. How does the molecular structure of this compound influence its reactivity in sol-gel processes?
Methodological Answer: The 1,3-substitution pattern on the benzene ring creates steric hindrance, slowing hydrolysis compared to 1,4-isomers. The triethoxysilyl groups hydrolyze sequentially to form siloxane (Si-O-Si) networks, enabling controlled crosslinking. Researchers should:
- Monitor hydrolysis kinetics using pH-dependent conductivity measurements .
- Adjust reaction conditions (e.g., acid/base catalysts) to modulate gelation time .
Advanced Research Questions
Q. How can researchers optimize the hydrolysis-condensation kinetics of this compound for tailored porosity in hybrid materials?
Methodological Answer: Porosity control requires balancing hydrolysis (Si-OEt cleavage) and condensation (Si-O-Si formation):
- Catalyst Selection: Acidic conditions (e.g., HCl) favor faster hydrolysis, while basic conditions (e.g., NH₃) accelerate condensation.
- Solvent Polarity: Polar solvents (e.g., ethanol) enhance hydrolysis but reduce crosslinking density.
- Additive Templating: Use surfactants (e.g., Pluronic P123) to template mesopores (2–50 nm). Post-synthesis, calcination removes templates, leaving ordered pores .
Q. What strategies resolve contradictions in surface area data between BET analysis and mercury porosimetry for materials derived from this compound?
Methodological Answer: Discrepancies arise from:
- BET Limitations: Assumes monolayer adsorption, underestimating micropore (<2 nm) contributions.
- Mercury Intrusion Artifacts: High pressure collapses mesopores, overestimating macropore (>50 nm) volumes.
Resolution: - Combine BET with Density Functional Theory (DFT) analysis for micropores.
- Validate with Small-Angle X-ray Scattering (SAXS) to assess pore uniformity .
Q. How can in-situ functionalization during co-condensation of this compound be achieved without disrupting mesopore ordering?
Methodological Answer: Co-condensation with functional silanes (e.g., mercaptopropyltrimethoxysilane) requires:
- Matching Hydrolysis Rates: Use silanes with similar hydrolysis kinetics (e.g., triethoxy vs. trimethoxy groups).
- Surfactant Compatibility: Non-ionic surfactants (e.g., Brij-56) minimize phase separation during templating.
- Post-Synthesis Analysis: Confirm functional group retention via X-ray Photoelectron Spectroscopy (XPS) and pore integrity via Transmission Electron Microscopy (TEM) .
特性
IUPAC Name |
triethoxy-(3-triethoxysilylphenyl)silane | |
---|---|---|
Source | PubChem | |
URL | https://pubchem.ncbi.nlm.nih.gov | |
Description | Data deposited in or computed by PubChem | |
InChI |
InChI=1S/C18H34O6Si2/c1-7-19-25(20-8-2,21-9-3)17-14-13-15-18(16-17)26(22-10-4,23-11-5)24-12-6/h13-16H,7-12H2,1-6H3 | |
Source | PubChem | |
URL | https://pubchem.ncbi.nlm.nih.gov | |
Description | Data deposited in or computed by PubChem | |
InChI Key |
MRBRVZDGOJHHFZ-UHFFFAOYSA-N | |
Source | PubChem | |
URL | https://pubchem.ncbi.nlm.nih.gov | |
Description | Data deposited in or computed by PubChem | |
Canonical SMILES |
CCO[Si](C1=CC(=CC=C1)[Si](OCC)(OCC)OCC)(OCC)OCC | |
Source | PubChem | |
URL | https://pubchem.ncbi.nlm.nih.gov | |
Description | Data deposited in or computed by PubChem | |
Molecular Formula |
C18H34O6Si2 | |
Source | PubChem | |
URL | https://pubchem.ncbi.nlm.nih.gov | |
Description | Data deposited in or computed by PubChem | |
DSSTOX Substance ID |
DTXSID70469322 | |
Record name | 1,3-Bis(triethoxysilyl)benzene | |
Source | EPA DSSTox | |
URL | https://comptox.epa.gov/dashboard/DTXSID70469322 | |
Description | DSSTox provides a high quality public chemistry resource for supporting improved predictive toxicology. | |
Molecular Weight |
402.6 g/mol | |
Source | PubChem | |
URL | https://pubchem.ncbi.nlm.nih.gov | |
Description | Data deposited in or computed by PubChem | |
CAS No. |
16067-99-5 | |
Record name | 1,3-Bis(triethoxysilyl)benzene | |
Source | EPA DSSTox | |
URL | https://comptox.epa.gov/dashboard/DTXSID70469322 | |
Description | DSSTox provides a high quality public chemistry resource for supporting improved predictive toxicology. | |
Synthesis routes and methods
Procedure details
Retrosynthesis Analysis
AI-Powered Synthesis Planning: Our tool employs the Template_relevance Pistachio, Template_relevance Bkms_metabolic, Template_relevance Pistachio_ringbreaker, Template_relevance Reaxys, Template_relevance Reaxys_biocatalysis model, leveraging a vast database of chemical reactions to predict feasible synthetic routes.
One-Step Synthesis Focus: Specifically designed for one-step synthesis, it provides concise and direct routes for your target compounds, streamlining the synthesis process.
Accurate Predictions: Utilizing the extensive PISTACHIO, BKMS_METABOLIC, PISTACHIO_RINGBREAKER, REAXYS, REAXYS_BIOCATALYSIS database, our tool offers high-accuracy predictions, reflecting the latest in chemical research and data.
Strategy Settings
Precursor scoring | Relevance Heuristic |
---|---|
Min. plausibility | 0.01 |
Model | Template_relevance |
Template Set | Pistachio/Bkms_metabolic/Pistachio_ringbreaker/Reaxys/Reaxys_biocatalysis |
Top-N result to add to graph | 6 |
Feasible Synthetic Routes
試験管内研究製品の免責事項と情報
BenchChemで提示されるすべての記事および製品情報は、情報提供を目的としています。BenchChemで購入可能な製品は、生体外研究のために特別に設計されています。生体外研究は、ラテン語の "in glass" に由来し、生物体の外で行われる実験を指します。これらの製品は医薬品または薬として分類されておらず、FDAから任何の医療状態、病気、または疾患の予防、治療、または治癒のために承認されていません。これらの製品を人間または動物に体内に導入する形態は、法律により厳格に禁止されています。これらのガイドラインに従うことは、研究と実験において法的および倫理的な基準の遵守を確実にするために重要です。