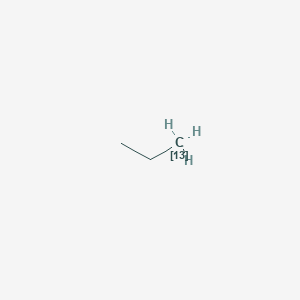
プロパン (1-13C)
概要
科学的研究の応用
Chemistry: It is used as a model compound to study the behavior of 5-HT3 receptor antagonists.
Biology: The compound is used to investigate the role of serotonin receptors in various biological processes.
Medicine: BRL-46470 has shown promise as an antiemetic and anxiolytic agent, although it has not been developed for medical use.
Industry: It is used in the development of new drugs targeting serotonin receptors
作用機序
BRL-46470は、セロトニン5-HT3受容体を選択的に拮抗することで効果を発揮します。この受容体は、中枢神経系と末梢神経系におけるシグナル伝達の伝達に関与しています。BRL-46470は、この受容体を遮断することにより、吐き気や不安を引き起こす神経応答の活性化を防ぐことができます .
生化学分析
Biochemical Properties
The analysis of the carbon-13 magnetic resonance spectrum of Propane (1-13C) yields one-, two-, and three-bond coupling constants between 13C nuclei and protons . These data serve as reference values in the experimental and theoretical study of substituent effects .
Molecular Mechanism
The molecular mechanism of Propane (1-13C) primarily involves its interactions with other molecules through its carbon-13 atom. The carbon-13 atom can be detected using nuclear magnetic resonance (NMR), allowing researchers to track the molecule’s interactions and transformations .
Temporal Effects in Laboratory Settings
The temporal effects of Propane (1-13C) in laboratory settings are primarily related to its stability. As a stable isotope, Propane (1-13C) does not undergo radioactive decay, making it suitable for long-term studies .
Metabolic Pathways
Propane (1-13C) can be involved in various metabolic pathways, just like regular propane. The carbon-13 label allows researchers to track the molecule’s metabolic transformations using NMR .
準備方法
BRL-46470の合成は、3,3-ジメチルインドリン-1-カルボニルクロリドとエンド-8-メチル-8-アザビシクロ[3.2.1]オクタン-3-アミンをトリエチルアミンを用いて縮合させ、続いて塩酸で塩化することによって行われます。中間体は、3-メチルインドールをメチルマグネシウムヨージド/ヨウ化メチルでメチル化し、続いて酢酸中白金上で水素化することにより3,3-ジメチルインドリンが得られます。これをホスゲンとトリエチルアミンで処理すると、中間体が生成されます .
化学反応の分析
BRL-46470は、次のような様々な化学反応を起こします。
酸化: 特定の条件下で酸化されて、異なる生成物を生成します。
還元: 一般的な還元剤を用いて還元できます。
置換: インドリン部位とアザビシクロ部位、特に置換反応を起こします。これらの反応で使用される一般的な試薬には、水素、白金、ホスゲン、トリエチルアミンなどがあります。
科学研究における用途
化学: 5-HT3受容体拮抗薬の挙動を研究するためのモデル化合物として使用されます。
生物学: 様々な生物学的プロセスにおけるセロトニン受容体の役割を調べるために使用されます。
医学: BRL-46470は、制吐薬および不安解薬として有望視されていますが、医療用として開発されていません。
類似化合物との比較
BRL-46470は、他の5-HT3受容体拮抗薬に比べて、強い不安解作用と副作用が少ないという点で独特です。類似の化合物には、以下のようなものがあります。
- ザトセトロン
- ベメセトロン
- トロパンセリン
- トロピセトロン
- グラニセトロン これらの化合物も5-HT3受容体拮抗薬として作用しますが、効力、作用時間、副作用のプロフィールが異なります .
特性
IUPAC Name |
(113C)propane | |
---|---|---|
Source | PubChem | |
URL | https://pubchem.ncbi.nlm.nih.gov | |
Description | Data deposited in or computed by PubChem | |
InChI |
InChI=1S/C3H8/c1-3-2/h3H2,1-2H3/i1+1 | |
Source | PubChem | |
URL | https://pubchem.ncbi.nlm.nih.gov | |
Description | Data deposited in or computed by PubChem | |
InChI Key |
ATUOYWHBWRKTHZ-OUBTZVSYSA-N | |
Source | PubChem | |
URL | https://pubchem.ncbi.nlm.nih.gov | |
Description | Data deposited in or computed by PubChem | |
Canonical SMILES |
CCC | |
Source | PubChem | |
URL | https://pubchem.ncbi.nlm.nih.gov | |
Description | Data deposited in or computed by PubChem | |
Isomeric SMILES |
CC[13CH3] | |
Source | PubChem | |
URL | https://pubchem.ncbi.nlm.nih.gov | |
Description | Data deposited in or computed by PubChem | |
Molecular Formula |
C3H8 | |
Source | PubChem | |
URL | https://pubchem.ncbi.nlm.nih.gov | |
Description | Data deposited in or computed by PubChem | |
Molecular Weight |
45.09 g/mol | |
Source | PubChem | |
URL | https://pubchem.ncbi.nlm.nih.gov | |
Description | Data deposited in or computed by PubChem | |
CAS No. |
17251-65-9 | |
Record name | 17251-65-9 | |
Source | European Chemicals Agency (ECHA) | |
URL | https://echa.europa.eu/information-on-chemicals | |
Description | The European Chemicals Agency (ECHA) is an agency of the European Union which is the driving force among regulatory authorities in implementing the EU's groundbreaking chemicals legislation for the benefit of human health and the environment as well as for innovation and competitiveness. | |
Explanation | Use of the information, documents and data from the ECHA website is subject to the terms and conditions of this Legal Notice, and subject to other binding limitations provided for under applicable law, the information, documents and data made available on the ECHA website may be reproduced, distributed and/or used, totally or in part, for non-commercial purposes provided that ECHA is acknowledged as the source: "Source: European Chemicals Agency, http://echa.europa.eu/". Such acknowledgement must be included in each copy of the material. ECHA permits and encourages organisations and individuals to create links to the ECHA website under the following cumulative conditions: Links can only be made to webpages that provide a link to the Legal Notice page. | |
Synthesis routes and methods I
Procedure details
Synthesis routes and methods II
Procedure details
Synthesis routes and methods III
Procedure details
Synthesis routes and methods IV
Procedure details
Retrosynthesis Analysis
AI-Powered Synthesis Planning: Our tool employs the Template_relevance Pistachio, Template_relevance Bkms_metabolic, Template_relevance Pistachio_ringbreaker, Template_relevance Reaxys, Template_relevance Reaxys_biocatalysis model, leveraging a vast database of chemical reactions to predict feasible synthetic routes.
One-Step Synthesis Focus: Specifically designed for one-step synthesis, it provides concise and direct routes for your target compounds, streamlining the synthesis process.
Accurate Predictions: Utilizing the extensive PISTACHIO, BKMS_METABOLIC, PISTACHIO_RINGBREAKER, REAXYS, REAXYS_BIOCATALYSIS database, our tool offers high-accuracy predictions, reflecting the latest in chemical research and data.
Strategy Settings
Precursor scoring | Relevance Heuristic |
---|---|
Min. plausibility | 0.01 |
Model | Template_relevance |
Template Set | Pistachio/Bkms_metabolic/Pistachio_ringbreaker/Reaxys/Reaxys_biocatalysis |
Top-N result to add to graph | 6 |
Feasible Synthetic Routes
Q1: How does propane (1-13C) help us understand the mechanism of propane aromatization on Zn-modified zeolites?
A: Propane (1-13C), specifically propane-1-13C, allows researchers to track the fate of the labeled carbon atom during the aromatization reaction using techniques like 13C MAS NMR [, ]. This helps decipher the reaction pathway and identify intermediate species. For example, studies have shown the formation of a propene adsorption complex with zinc sites (π-complex) and σ-allylzinc species during the conversion of propane to aromatics [].
Q2: What are the undesirable reaction pathways observed during propane aromatization on Zn-modified zeolites, and how does propane (1-13C) help study them?
A: Besides the desired aromatization, propane can undergo hydrogenolysis, leading to the formation of methane and ethane []. Using propane-1-13C and propane-2-13C, combined with kinetic modeling based on in situ 1H MAS NMR measurements, researchers can distinguish between these pathways and analyze the role of Brønsted acid sites and Zn sites in the hydrogenolysis process [].
Q3: What insights can be obtained from studying the interaction of propane (1-13C) with different Zn species present in zeolites?
A: Research suggests that the nature of Zn species in zeolites significantly influences the catalytic activity and selectivity for propane aromatization and hydrogenolysis []. Using propane (1-13C) as a probe molecule in conjunction with spectroscopic techniques allows for a deeper understanding of how different Zn species interact with propane, influencing reaction pathways and product distribution. This information is crucial for designing more efficient and selective catalysts for propane conversion.
試験管内研究製品の免責事項と情報
BenchChemで提示されるすべての記事および製品情報は、情報提供を目的としています。BenchChemで購入可能な製品は、生体外研究のために特別に設計されています。生体外研究は、ラテン語の "in glass" に由来し、生物体の外で行われる実験を指します。これらの製品は医薬品または薬として分類されておらず、FDAから任何の医療状態、病気、または疾患の予防、治療、または治癒のために承認されていません。これらの製品を人間または動物に体内に導入する形態は、法律により厳格に禁止されています。これらのガイドラインに従うことは、研究と実験において法的および倫理的な基準の遵守を確実にするために重要です。