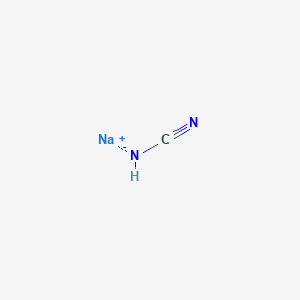
Sodium hydrogencyanamide
概要
説明
Sodium hydrogencyanamide (CAS: 17292-62-5), also known as sodium cyanamide, is an inorganic compound with the molecular formula CHN₂Na and a molecular weight of 64.02 g/mol . It is characterized by a high melting point (>360°C) and boiling point (258.5°C at 760 mmHg), with a flashpoint of 1.2°C . Industrially, it is synthesized from calcium cyanamide (CaCN₂) via reaction with sodium hydroxide or sodium sulfate .
準備方法
Synthetic Routes and Reaction Conditions: Sodium hydrogencyanamide can be synthesized through several methods. One common method involves the reaction of calcium cyanamide with sodium hydroxide. The reaction proceeds as follows: [ \text{CaCN2} + 2 \text{NaOH} \rightarrow \text{Na2CN2} + \text{Ca(OH)2} ] This reaction is typically carried out at elevated temperatures to ensure complete conversion.
Industrial Production Methods: In industrial settings, sodium cyanamide is often produced by the hydrolysis of calcium cyanamide. The process involves the following steps:
Addition of Lime Nitrogen: Lime nitrogen (calcium cyanamide) is added to a sodium sulfate aqueous solution.
Reaction: The lime nitrogen reacts with sodium sulfate to generate sodium hydrogen cyanamide.
Filtration: The mixture is filtered to remove calcium hydroxide and part of the sodium hydrogen sulfate.
Neutralization: Sodium hydrogen cyanamide is neutralized using hydrochloric acid.
Concentration: The solution is concentrated under reduced pressure to remove sodium chloride and sodium hydrogen sulfate.
化学反応の分析
Types of Reactions: Sodium hydrogencyanamide undergoes various chemical reactions, including:
Oxidation: this compound can be oxidized to form sodium cyanate.
Reduction: It can be reduced to form sodium amide.
Substitution: this compound can undergo substitution reactions with various electrophiles.
Common Reagents and Conditions:
Oxidation: Hydrogen peroxide (H2O2) is commonly used as an oxidizing agent.
Reduction: Sodium metal or lithium aluminum hydride (LiAlH4) can be used as reducing agents.
Substitution: Electrophiles such as alkyl halides or acyl chlorides are used in substitution reactions.
Major Products:
Oxidation: Sodium cyanate (NaOCN)
Reduction: Sodium amide (NaNH2)
Substitution: Various substituted cyanamides depending on the electrophile used.
科学的研究の応用
Agricultural Applications
Sodium hydrogencyanamide is primarily utilized in agriculture to enhance the growth and yield of various crops. The compound is effective in breaking dormancy in fruit trees and promoting flowering, which can lead to earlier harvests.
Bud Dormancy Release
Research indicates that this compound facilitates the release of bud dormancy in several perennial species, including:
- Peach (Prunus persica)
- Apple
- Grapevine
- Sweet Cherry
In these species, the application of this compound has been shown to affect gene expression related to floral regulation and antioxidant metabolism, thereby inducing bud break at controlled times .
Table 1: Effects of this compound on Bud Dormancy Release
Crop Type | Application Rate | Observed Effects |
---|---|---|
Peach | 2% | Enhanced bud break and flowering |
Apple | Varies | Increased fruit yield |
Grapevine | Varies | Earlier flowering and harvest |
Sweet Cherry | 2% | Improved timing of harvest |
Mechanistic Insights
The mechanism by which this compound operates involves the generation of sub-lethal oxidative stress, which triggers physiological changes within the plant. Studies have demonstrated that treatment with this compound leads to increased levels of hydrogen peroxide and alterations in phytohormone levels, including a decrease in abscisic acid (ABA) and an increase in ethylene production .
Toxicological Studies
While this compound has beneficial applications, it is also associated with toxicity risks. Case studies highlight instances of poisoning from accidental or intentional ingestion.
Case Study: Acute Poisoning Incidents
A notable case involved a young male who ingested a significant amount of this compound (DORMEX®) with suicidal intent. Despite intensive care management, the patient succumbed due to severe metabolic acidosis and shock . This underscores the need for caution when handling this compound.
Table 2: Summary of Poisoning Cases Related to this compound
Case Study Reference | Patient Outcome | Symptoms Observed |
---|---|---|
Fatal | Stupor, hypotension, metabolic acidosis | |
Fatal | Severe vomiting, shock | |
Fully recovered | Flushing, nausea, dyspnoea |
Research and Development
Ongoing research continues to explore new formulations and applications for this compound. Recent studies have focused on developing solid formulations that enhance its herbicidal properties against common crop weeds . These advancements aim to improve agricultural efficiency while minimizing environmental impact.
作用機序
The mechanism of action of sodium cyanamide involves its ability to inhibit certain enzymes. For example, in the treatment of alcoholism, sodium cyanamide inhibits aldehyde dehydrogenase, leading to the accumulation of acetaldehyde in the body. This results in unpleasant symptoms when alcohol is consumed, thereby discouraging alcohol intake .
類似化合物との比較
Structural and Functional Differences
Toxicity Profiles
- This compound : Causes hepatotoxicity and disulfiram-like syndrome upon prolonged exposure .
- Sodium Cyanide (NaCN) : Rapidly lethal via inhibition of cytochrome c oxidase .
- Calcium Cyanamide : Low acute toxicity but irritant to mucous membranes .
Industrial and Agricultural Roles
- This compound : Specialized in bud break induction and pharmaceutical intermediates.
- Sodium Cyanide : Critical in gold mining but heavily regulated due to environmental risks .
- Sodium Dicyanamide : Niche use in high-temperature trimerization reactions for melamine production .
Research Findings and Data
- Antiproliferative Activity : Guanidine derivatives synthesized from this compound (e.g., compounds 12 and 15) showed superior cytotoxicity against Colo 205 and Colo 320 cancer cells compared to cisplatin .
- Thermal Behavior : Sodium dicyanamide trimerizes to trisodium tricyanmelaminate at high temperatures, a reaction absent in this compound .
- Environmental Impact : Sodium cyanide poses significant ecological risks, whereas this compound’s degradation products (e.g., urea) are less hazardous .
生物活性
Sodium hydrogencyanamide, commonly known as hydrogen cyanamide, is a compound extensively used in agriculture, particularly as a plant growth regulator. Its primary application is to break dormancy in perennial crops, facilitating earlier flowering and fruiting. This article explores the biological activity of this compound, focusing on its mechanisms of action, effects on plant physiology, and relevant case studies.
Hydrogen cyanamide acts primarily by inducing oxidative stress in plant tissues, which triggers various physiological responses that lead to the release from dormancy. The key mechanisms include:
- Reactive Oxygen Species (ROS) Production : Treatment with hydrogen cyanamide results in a rapid accumulation of ROS, which plays a crucial role in signaling pathways associated with dormancy release. Studies have shown that higher levels of hydrogen peroxide (H₂O₂) and nitric oxide (NO) correlate with increased budbreak rates in grapevines treated with hydrogen cyanamide compared to controls .
- Phytohormonal Changes : The application of hydrogen cyanamide alters the levels of several phytohormones. For instance, it has been observed to decrease abscisic acid (ABA) levels while increasing cytokinin and ethylene levels, which are essential for promoting growth and development .
- Gene Expression Modulation : Transcriptomic analyses reveal that hydrogen cyanamide treatment leads to significant changes in gene expression related to bud development and stress responses. Key pathways affected include those related to jasmonates and cytokinins .
Effects on Plant Physiology
Hydrogen cyanamide has been shown to have various effects on plant physiology:
- Bud Dormancy Release : In perennial species such as grapevines, peach, and apple trees, hydrogen cyanamide effectively breaks dormancy, allowing for synchronized flowering and fruiting. For example, grapevine buds treated with hydrogen cyanamide showed budbreak rates of up to 95% compared to 0% in untreated controls .
- Antioxidant Activity : The compound influences antioxidant metabolism by increasing the ratio of reduced to oxidized glutathione and elevating proline levels, which are vital for stress tolerance .
- Impact on Yield : By controlling the timing of budbreak, hydrogen cyanamide can lead to improved yields in economically important crops like sweet cherries and grapes, where timely harvest is crucial .
Case Study 1: Grape Bud Dormancy
A study conducted on grapevines demonstrated that treatment with 12.5 mM hydrogen cyanamide significantly enhanced budbreak rates compared to control groups. The study utilized microarray analysis to assess gene expression changes post-treatment, revealing that the combined treatment of pruning and hydrogen cyanamide resulted in the highest expression alteration among treatments .
Treatment | Budbreak Rate (%) |
---|---|
Control | 0 |
Pruning | 33 |
Hydrogen Cyanamide | 53 |
Pruning + Hydrogen Cyanamide | 95 |
Case Study 2: Acute Poisoning Incident
Despite its agricultural benefits, this compound poses risks if misused. A case report documented acute poisoning from ingestion of hydrogen cyanamide, leading to severe metabolic acidosis and shock. This incident highlights the importance of safety measures when handling this compound .
Q & A
Basic Research Questions
Q. What are the standard methods for synthesizing sodium hydrogencyanamide, and how can purity be validated?
this compound (CHN₂Na) is typically synthesized by reacting cyanamide with sodium hydroxide under controlled stoichiometric conditions. To ensure purity, researchers should employ:
- Titration (e.g., acid-base titration to quantify residual NaOH).
- Spectroscopic techniques (e.g., FT-IR to confirm functional groups like –CN and –NH₂).
- Thermogravimetric analysis (TGA) to assess thermal stability and decomposition patterns .
- Elemental analysis to verify stoichiometric ratios of C, H, N, and Na .
Q. Which analytical techniques are most effective for characterizing this compound in solution-phase studies?
- High-Performance Liquid Chromatography (HPLC) with UV detection (λ = 200–220 nm) to quantify dissolved species.
- Nuclear Magnetic Resonance (NMR) (¹³C and ¹⁵N isotopes) to track structural changes in aqueous environments.
- pH-dependent solubility assays to evaluate stability across biological or environmental conditions .
Q. How can researchers mitigate risks when handling this compound in laboratory settings?
- Use glove boxes or fume hoods to prevent inhalation/contact.
- Implement PPE protocols : nitrile gloves, lab coats, and safety goggles.
- Establish emergency protocols : immediate rinsing with water for skin/eye exposure and neutralization of spills with weak acids (e.g., acetic acid) .
Advanced Research Questions
Q. What experimental designs are optimal for studying this compound’s role in plant growth regulation?
- Controlled hydroponic studies with variable concentrations (e.g., 0.1–10 mM) to assess dose-dependent effects on germination rates.
- Transcriptomic analysis (RNA sequencing) to identify gene expression changes in model plants (e.g., Arabidopsis thaliana).
- Comparative studies with alternative growth regulators (e.g., gibberellins) to evaluate efficacy and mechanistic differences .
Q. How can contradictory findings in this compound toxicity studies be resolved?
- Conduct systematic reviews/meta-analyses to identify confounding variables (e.g., species-specific metabolic pathways).
- Use in vitro hepatocyte models to isolate cytotoxic mechanisms (e.g., oxidative stress markers like glutathione depletion) .
- Validate findings via cross-laboratory reproducibility studies with standardized protocols (e.g., ISO 10993 for biocompatibility testing) .
Q. What novel applications of this compound are emerging in synthetic chemistry?
- Explore its use as a nitrogen donor in heterocyclic compound synthesis (e.g., triazoles or tetrazoles) via [2+3] cycloaddition reactions.
- Optimize reaction conditions (solvent polarity, temperature) using Design of Experiments (DoE) to maximize yield and selectivity .
Q. How can computational modeling enhance understanding of this compound’s reactivity?
- Apply Density Functional Theory (DFT) to simulate reaction pathways (e.g., nucleophilic attack at the cyanamide group).
- Validate models with experimental kinetic data (e.g., Arrhenius plots for decomposition rates) .
Q. Methodological Resources
- Data Analysis : Use tools like R or Python for dose-response curve fitting and ANOVA to compare treatment groups .
- Literature Review : Prioritize primary sources from databases like PubMed or SciFinder, avoiding non-peer-reviewed platforms .
- Ethical Compliance : Adhere to institutional guidelines for chemical waste disposal and toxicity testing in biological studies .
特性
IUPAC Name |
sodium;cyanoazanide | |
---|---|---|
Source | PubChem | |
URL | https://pubchem.ncbi.nlm.nih.gov | |
Description | Data deposited in or computed by PubChem | |
InChI |
InChI=1S/CHN2.Na/c2-1-3;/h2H;/q-1;+1 | |
Source | PubChem | |
URL | https://pubchem.ncbi.nlm.nih.gov | |
Description | Data deposited in or computed by PubChem | |
InChI Key |
MBEGFNBBAVRKLK-UHFFFAOYSA-N | |
Source | PubChem | |
URL | https://pubchem.ncbi.nlm.nih.gov | |
Description | Data deposited in or computed by PubChem | |
Canonical SMILES |
C(#N)[NH-].[Na+] | |
Source | PubChem | |
URL | https://pubchem.ncbi.nlm.nih.gov | |
Description | Data deposited in or computed by PubChem | |
Molecular Formula |
CHN2Na | |
Source | PubChem | |
URL | https://pubchem.ncbi.nlm.nih.gov | |
Description | Data deposited in or computed by PubChem | |
DSSTOX Substance ID |
DTXSID9051791 | |
Record name | Sodium cyanamide | |
Source | EPA DSSTox | |
URL | https://comptox.epa.gov/dashboard/DTXSID9051791 | |
Description | DSSTox provides a high quality public chemistry resource for supporting improved predictive toxicology. | |
Molecular Weight |
64.022 g/mol | |
Source | PubChem | |
URL | https://pubchem.ncbi.nlm.nih.gov | |
Description | Data deposited in or computed by PubChem | |
CAS No. |
17292-62-5 | |
Record name | Cyanamide, sodium salt (1:1) | |
Source | ChemIDplus | |
URL | https://pubchem.ncbi.nlm.nih.gov/substance/?source=chemidplus&sourceid=0017292625 | |
Description | ChemIDplus is a free, web search system that provides access to the structure and nomenclature authority files used for the identification of chemical substances cited in National Library of Medicine (NLM) databases, including the TOXNET system. | |
Record name | Cyanamide, sodium salt (1:1) | |
Source | EPA Chemicals under the TSCA | |
URL | https://www.epa.gov/chemicals-under-tsca | |
Description | EPA Chemicals under the Toxic Substances Control Act (TSCA) collection contains information on chemicals and their regulations under TSCA, including non-confidential content from the TSCA Chemical Substance Inventory and Chemical Data Reporting. | |
Record name | Sodium cyanamide | |
Source | EPA DSSTox | |
URL | https://comptox.epa.gov/dashboard/DTXSID9051791 | |
Description | DSSTox provides a high quality public chemistry resource for supporting improved predictive toxicology. | |
Record name | Sodium cyanamidate | |
Source | European Chemicals Agency (ECHA) | |
URL | https://echa.europa.eu/substance-information/-/substanceinfo/100.037.550 | |
Description | The European Chemicals Agency (ECHA) is an agency of the European Union which is the driving force among regulatory authorities in implementing the EU's groundbreaking chemicals legislation for the benefit of human health and the environment as well as for innovation and competitiveness. | |
Explanation | Use of the information, documents and data from the ECHA website is subject to the terms and conditions of this Legal Notice, and subject to other binding limitations provided for under applicable law, the information, documents and data made available on the ECHA website may be reproduced, distributed and/or used, totally or in part, for non-commercial purposes provided that ECHA is acknowledged as the source: "Source: European Chemicals Agency, http://echa.europa.eu/". Such acknowledgement must be included in each copy of the material. ECHA permits and encourages organisations and individuals to create links to the ECHA website under the following cumulative conditions: Links can only be made to webpages that provide a link to the Legal Notice page. | |
Retrosynthesis Analysis
AI-Powered Synthesis Planning: Our tool employs the Template_relevance Pistachio, Template_relevance Bkms_metabolic, Template_relevance Pistachio_ringbreaker, Template_relevance Reaxys, Template_relevance Reaxys_biocatalysis model, leveraging a vast database of chemical reactions to predict feasible synthetic routes.
One-Step Synthesis Focus: Specifically designed for one-step synthesis, it provides concise and direct routes for your target compounds, streamlining the synthesis process.
Accurate Predictions: Utilizing the extensive PISTACHIO, BKMS_METABOLIC, PISTACHIO_RINGBREAKER, REAXYS, REAXYS_BIOCATALYSIS database, our tool offers high-accuracy predictions, reflecting the latest in chemical research and data.
Strategy Settings
Precursor scoring | Relevance Heuristic |
---|---|
Min. plausibility | 0.01 |
Model | Template_relevance |
Template Set | Pistachio/Bkms_metabolic/Pistachio_ringbreaker/Reaxys/Reaxys_biocatalysis |
Top-N result to add to graph | 6 |
Feasible Synthetic Routes
試験管内研究製品の免責事項と情報
BenchChemで提示されるすべての記事および製品情報は、情報提供を目的としています。BenchChemで購入可能な製品は、生体外研究のために特別に設計されています。生体外研究は、ラテン語の "in glass" に由来し、生物体の外で行われる実験を指します。これらの製品は医薬品または薬として分類されておらず、FDAから任何の医療状態、病気、または疾患の予防、治療、または治癒のために承認されていません。これらの製品を人間または動物に体内に導入する形態は、法律により厳格に禁止されています。これらのガイドラインに従うことは、研究と実験において法的および倫理的な基準の遵守を確実にするために重要です。