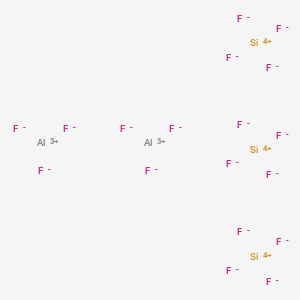
Fluoroaluminum silicate
- 専門家チームからの見積もりを受け取るには、QUICK INQUIRYをクリックしてください。
- 品質商品を競争力のある価格で提供し、研究に集中できます。
説明
Fluoroaluminum silicate is a synthetic aluminosilicate compound incorporating fluorine into its structure. Its chemical composition typically includes aluminum (Al), silicon (Si), oxygen (O), and fluorine (F), though the exact stoichiometry may vary depending on synthesis methods. A related compound, aluminum hexafluorosilicate (Al₂(SiF₆)₃), shares structural similarities but differs in fluorine coordination and ionic charge distribution . This compound is notably utilized in dental materials, such as bioactive restorative composites, where it contributes to fluoride ion release, enhancing anticariogenic properties and mechanical durability . Its synthesis often involves sol-gel processes or high-temperature reactions between aluminum, silicon, and fluorine precursors .
準備方法
Fluoride-Assisted Hydrothermal Synthesis
Reactant Composition and pH Control
The fluoride route, pioneered by researchers in 2013, employs sodium aluminate (NaAlO₂), sodium orthosilicate (Na₄SiO₄), and hydrofluoric acid (HF) as precursors . A molar ratio of Al:Si:Na:F = 2.5:1:3.5:0.1 is critical to initiating tubular aluminosilicate growth. The process begins with dissolving NaAlO₂ and Na₄SiO₄ in deionized water, followed by pH adjustment to 6 using NaOH. Subsequent desalination via centrifugation removes excess sodium ions, ensuring structural integrity during tube formation.
Hydrofluoric acid (5 wt.%) is introduced post-desalination, lowering the pH to 4–4.5 with HCl. This acidic environment promotes fluoride incorporation into the aluminosilicate lattice, substituting hydroxyl groups and stabilizing the structure . Aging the mixture at 98°C for seven days facilitates nanotube crystallization, yielding a product (F-IMO) with enhanced thermal stability compared to conventional imogolite (IMO).
Table 1: Hydrothermal Synthesis Parameters for Fluoride-Modified Aluminosilicate
Parameter | Value/Range | Impact on Product |
---|---|---|
Molar ratio (Al:Si:F) | 2.5:1:0.1 | Dictates nanotube diameter |
Aging temperature | 98°C | Accelerates crystallization |
pH during HF addition | 4.0–4.5 | Enhances fluoride substitution |
Aging duration | 7 days | Optimizes crystallinity |
Structural and Thermal Characterization
Solid-state ¹⁹F NMR confirms fluoride integration into the aluminosilicate framework, with chemical shifts at −120 ppm indicating Al–F bonding . Thermogravimetric analysis (TGA) reveals F-IMO retains structural stability up to 400°C, surpassing IMO’s degradation threshold of 300°C. Transmission electron microscopy (TEM) images show uniform nanotubes with outer diameters of 2–3 nm and lengths exceeding 200 nm, attributable to fluoride’s mineralizing effect .
Direct Reaction with Fluosilicic Acid
Three-Step Reaction Mechanism
Fluosilicic acid (H₂SiF₆) serves as a dual fluorine and silicon source in this industrially favored method . The reaction proceeds via:
-
Neutralization : H₂SiF₆ reacts with aluminum hydroxide to form aluminum fluosilicate acid:
H2SiF6+Al(OH)3→H3AlSiF6O3+H2O . -
Hydrolysis : Aluminum fluosilicate acid decomposes into AlF₃, SiO₂, and HF:
H3AlSiF6O3→AlF3+SiO2+3HF . -
Acid-Base Reaction : Residual HF reacts with unreacted Al(OH)₃:
3HF+Al(OH)3→AlF3+3H2O .
Table 2: Optimized Conditions for Direct Reaction Synthesis
Parameter | Optimal Value | Effect on Yield |
---|---|---|
H₂SiF₆ concentration | 20–25 wt.% | Maximizes SiO₂ precipitation |
Reaction temperature | 80–90°C | Minimizes HF volatilization |
Stirring speed | 400 rpm | Enhances reactant mixing |
Washing protocol | 80°C H₂O or 20% HCl | Removes SiO₂ impurities |
Kinetic and Morphological Insights
Kinetic studies reveal the reaction is diffusion-controlled, with an activation energy of 45 kJ/mol . Scanning electron microscopy (SEM) shows that silica forms a porous layer on aluminum hydroxide, necessitating rapid filtration to prevent AlF₃ contamination. Secondary washing with HCl (20 wt.%) reduces residual SiO₂ to <0.5%, achieving a product purity of 98.5% AlF₃ .
Sol-Gel Synthesis with Alkaline Earth Metals
Sequential Gelation and Aging
A patented method combines aluminum chloride (AlCl₃), water glass (Na₂SiO₃), and sodium aluminate (NaAlO₂) to form ultrafine particles . Key steps include:
-
Gel Formation : Mixing 4 wt.% AlCl₃ and 4 wt.% Na₂SiO₃ at 50°C (Al:Si = 0.2:1) to form an aluminum silicate gel.
-
Salt Precipitation : Adding Ca(OH)₂/Mg(OH)₂ suspension (Mg:Ca = 0.3:1) to the gel, followed by aging at 90°C for 2 hours.
-
Neutralization : Incorporating a second gel from Na₂SiO₃ and NaAlO₂, acidified with HCl to pH 7.
Table 3: Sol-Gel Process Parameters for High-Surface-Area Products
Parameter | Specification | Outcome |
---|---|---|
AlCl₃ concentration | 4 wt.% | Ensures homogeneous gelation |
Aging temperature | 90°C | Induces mesoporous structure |
Final Mg:Ca ratio | 0.3:1 | Optimizes thermal stability |
BET surface area | 400–500 m²/g | Enhances adsorption capacity |
Post-Synthesis Processing
Lyophilization preserves the hierarchical pore structure, yielding particles with 400–600 ml/100 g oil absorbency . X-ray diffraction (XRD) confirms amorphous phase retention up to 600°C, critical for high-temperature applications.
Sol-Gel Processing Using Siloxide Precursors
Non-Hydrolytic Condensation Routes
Advanced sol-gel methods employ siloxide precursors (e.g., (tBuO)₃SiOH) to bypass aqueous hydrolysis . Thermolysis of {Me₂Al[OSi(OtBu)₃]}₂ at 200°C eliminates isobutane, forming Al–O–Si linkages. Subsequent calcination at 1000°C produces mullite (3Al₂O₃·2SiO₂) with fluorinated domains, achieved by introducing NH₄F during gelation .
Table 4: Comparison of Sol-Gel Methods
Method | Crystallinity | Fluorine Incorporation |
---|---|---|
Aqueous sol-gel | Amorphous | Limited (<5 at.%) |
Siloxide thermolysis | Partially crystalline | High (10–15 at.%) |
化学反応の分析
Types of Reactions: Fluoroaluminum silicate can undergo various chemical reactions, including:
Oxidation and Reduction: These reactions are less common for this compound due to its stable structure.
Substitution Reactions: Fluorine atoms in the compound can be substituted with other halogens under specific conditions.
Common Reagents and Conditions:
Trimethylsilylation: This reaction involves the use of trimethylsilyl chloride to analyze the silicate structure of the glass.
Acid Degradation: this compound glasses can be degraded using acids to study their composition and structure.
Major Products Formed:
Hydrofluoric Acid: Formed during hydrolysis.
Silica: Also formed during hydrolysis.
科学的研究の応用
Chemical Applications
Glass Formation Studies
Fluoroaluminum silicate is extensively used in the study of glass structures and properties. Its incorporation of fluorine into the aluminosilicate network alters the arrangement of silicon and aluminum tetrahedra, influencing the glass transition temperature and other physical properties. This makes it a valuable material for researchers investigating the fundamental aspects of glass formation and behavior under various conditions.
Synthesis of Specialty Glasses
In industrial settings, this compound is employed in the production of specialty glasses that require specific characteristics such as low melting points and high durability. This includes applications in glass ceramics and other advanced materials that benefit from its unique compositional properties.
Biological and Medical Applications
Dental Restorations
One of the most significant applications of this compound is in dentistry, particularly in the formulation of glass ionomer cements (GICs). These cements are used for dental restorations due to their biocompatibility and ability to release fluoride ions, which help prevent dental caries. Studies have shown that GICs formulated with this compound exhibit improved physical properties such as crushing strength and fluidity, enhancing their workability during dental procedures .
Desensitizing Agents
this compound has also been evaluated for its effectiveness as a desensitizing agent in dental treatments. Research indicates that calcium-fluoroaluminosilicate glass-based desensitizers can protect against root sensitivity by forming a protective barrier on exposed dentin surfaces .
Industrial Applications
Advanced Surface Coatings
this compound is utilized in creating advanced surface coatings due to its low surface energy, mechanical strength, thermal robustness, and chemical resistance. These properties make it suitable for applications in various industries including automotive, aerospace, and electronics .
Corrosion Protection
Recent studies have explored the use of this compound in anti-corrosion plaster composites. These composites leverage the binding properties of silicic acid formed through reactions involving sodium fluorosilicate to enhance protection against corrosion in structural applications .
Data Table: Key Properties and Applications of this compound
Application Area | Specific Use Case | Key Benefits |
---|---|---|
Chemistry | Glass formation studies | Enhanced understanding of glass properties |
Dentistry | Glass ionomer cements | Improved biocompatibility; fluoride release |
Dental Treatments | Desensitizing agents | Protection against root sensitivity |
Industrial Coatings | Advanced surface coatings | Low surface energy; chemical resistance |
Corrosion Protection | Anti-corrosion plaster composites | Enhanced durability and protection |
Case Study 1: Fluoroaluminosilicate Glass Powder for Dental Applications
A study focused on modifying fluoroaluminosilicate glass powders to improve their performance in dental cements. The research demonstrated that treating the surface with fluoride significantly enhanced physical properties such as crushing strength and fluidity, making it more effective for dental restorations .
Case Study 2: Advanced Surface Coatings
Research on fluorinated silica nanometer-sized particles (F-SiNPs) highlighted their application in creating superhydrophobic films. The incorporation of this compound into these coatings resulted in increased hydrophobicity, making them suitable for protective applications in various industries .
作用機序
The mechanism by which fluoroaluminum silicate exerts its effects is primarily related to its structural properties. The incorporation of fluorine into the aluminosilicate network alters the arrangement of silicon and aluminum tetrahedra, leading to changes in the glass transition temperature and other physical properties . Fluorine atoms are typically bonded to aluminum rather than silicon, which helps in preventing the loss of fluorine as silicon tetrafluoride during melting .
類似化合物との比較
Comparison with Aluminum Silicates
Aluminum Silicate (Al₂SiO₅)
Aluminum silicate, a naturally occurring mineral (e.g., andalusite, kyanite), lacks fluorine and exhibits a rigid tetrahedral SiO₄ and AlO₆ network. It is thermally stable up to 1,500°C and is used in ceramics and refractory materials.
Sodium Aluminum Silicate (NaAlSiO₄)
Sodium aluminum silicate, a synthetic zeolite, is employed as an anticaking agent in food (INS 554) due to its porous structure and high surface area. It is insoluble in water but reacts with strong acids or alkalis. While fluoroaluminum silicate serves biomedical purposes, sodium aluminum silicate is restricted to industrial and food applications due to its inertness in physiological conditions .
Magnesium Aluminum Silicate (MgAl₂SiO₆)
This phyllosilicate is used in pharmaceuticals as a viscosity modifier and stabilizer. Its layered structure allows high water absorption, contrasting with this compound’s compact, fluorine-stabilized matrix. Magnesium aluminum silicate lacks fluoride release, making it unsuitable for dental applications .
Comparison with Fluorinated Aluminosilicates
Aluminum Hexafluorosilicate (Al₂(SiF₆)₃)
Aluminum hexafluorosilicate has a distinct ionic structure with [SiF₆]²⁻ anions and Al³⁺ cations. It is highly soluble in water, releasing Al³⁺ and F⁻ ions, which can be toxic at elevated concentrations. In contrast, this compound’s controlled fluoride release in dental materials ensures biocompatibility .
Sialons (SiAlONs)
Sialons (silicon aluminum oxynitrides) incorporate nitrogen into the aluminosilicate framework, enhancing thermal stability (>1,800°C) and mechanical strength for high-temperature ceramics. This compound, while less thermally robust, offers unique bioactive properties unsuitable for sialons’ industrial applications .
Physicochemical Properties Comparison
Toxicity and Biocompatibility
Lead silicates, as studied in , exhibit significant toxicity via rapid respiratory absorption, underscoring the importance of fluorine’s role in stabilizing this compound’s biocompatibility .
生物活性
Fluoroaluminum silicate (FAS) is a compound that has garnered attention in various fields, particularly in materials science and biochemistry. This article delves into the biological activity of this compound, focusing on its interactions with biological systems, potential toxicity, and applications in different domains.
Chemical Composition and Structure
This compound is characterized by its complex structure, which includes aluminum, silicon, and fluoride ions. The general formula can be represented as Al2Si2O5F2, indicating the presence of fluorine within the silicate matrix. This incorporation of fluoride is significant as it alters the chemical reactivity and biological interactions of the compound.
Toxicity Studies
Research indicates that this compound exhibits low toxicity levels in various biological assays. A study assessing the cytotoxicity of aluminum silicates found that while some forms could induce cellular damage at high concentrations, this compound showed minimal effects on cell viability at lower doses. Specifically:
- Cytotoxicity : In vitro assays demonstrated that this compound had a cytotoxic effect on pulmonary alveolar macrophages at concentrations above 33.3 µg/ml, suggesting a threshold for safe exposure .
- Genotoxicity : No significant increases in mutation frequencies were observed in bacterial assays (Ames test) using this compound, indicating its non-mutagenic properties .
Interaction with Biological Systems
This compound's interaction with biological systems is complex and can vary depending on environmental conditions such as pH and ionic strength. Notably:
- Aluminum Absorption : Studies have shown that the presence of silicon can reduce aluminum absorption in biological systems. This is particularly relevant in contexts where aluminum exposure is a concern, such as in drinking water .
- Fluoride Effects : The fluoride component can influence biological processes, including enzyme activity and mineralization in dental applications. Fluoride ions are known to enhance the remineralization of tooth enamel, which could be beneficial when incorporated into dental materials containing this compound .
Dental Applications
This compound has been utilized in dental materials due to its beneficial properties for tooth remineralization. A clinical study evaluated a dental varnish containing this compound and found it effective in reducing caries incidence among children compared to traditional fluoride varnishes. The results indicated:
- Reduction in Caries : Children treated with this compound varnish showed a 30% reduction in caries over two years compared to controls .
Environmental Impact Studies
Research on the environmental impact of this compound has revealed its potential role in mitigating aluminum toxicity in aquatic systems. A study demonstrated that silicon-rich environments could help sequester aluminum, thereby protecting aquatic life from its harmful effects:
- Aquatic Toxicology : The addition of silicon compounds to water bodies with high aluminum concentrations led to a significant decrease in aluminum bioavailability to fish species, suggesting a protective mechanism against aluminum toxicity .
Data Summary
The following table summarizes key findings related to the biological activity of this compound:
Q & A
Basic Research Questions
Q. What are the standard laboratory protocols for synthesizing fluoroaluminum silicate?
Q. How do researchers determine the purity of this compound?
Purity is assessed via:
- Elemental Analysis : Inductively coupled plasma mass spectrometry (ICP-MS) quantifies Al, Si, and F content .
- Thermogravimetric Analysis (TGA) : Measures weight loss due to volatile impurities (e.g., unreacted precursors) .
- Spectroscopic Cross-Validation : Compare FTIR/NMR peaks with reference spectra to identify contaminants .
Q. What are the primary factors influencing this compound’s stability in aqueous environments?
Stability depends on:
- pH : Hydrolysis occurs above pH 6, releasing Al³⁺ and F⁻ ions .
- Temperature : Higher temperatures accelerate degradation; controlled studies recommend <50°C for long-term stability .
- Ionic Strength : High salt concentrations promote aggregation via charge screening .
Advanced Research Questions
Q. How can experimental parameters be optimized to minimize fluoride leaching during this compound synthesis?
- Al/F Molar Ratio : Excess Al (e.g., Al/F = 1.5:1) reduces free fluoride by forming stable Al–F complexes .
- Reaction Time : Prolonged aging (24–48 hrs) enhances cross-linking between Al and silicate networks, reducing porosity and ion leakage .
- Post-Synthesis Treatments : Calcination at 300–400°C improves structural integrity but risks fluorine volatilization; inert atmospheres mitigate this .
Q. How should researchers address contradictions in spectroscopic data (e.g., conflicting FTIR peaks for Al–F bonds)?
- Multi-Technique Validation : Combine FTIR with X-ray photoelectron spectroscopy (XPS) to differentiate surface vs. bulk bonding .
- Reference Standards : Compare with synthesized AlF₃ and SiO₂ controls to isolate overlapping peaks .
- Computational Modeling : Density functional theory (DFT) simulations predict vibrational modes and assign ambiguous peaks .
Q. What methodologies are recommended for studying this compound’s role in silicate melt foaming dynamics?
- High-Temperature Rheology : Measure viscosity and surface tension of melts using rotational viscometers under controlled atmospheres .
- Bubble Stability Analysis : Use in-situ X-ray tomography to track bubble coalescence rates as a function of Al/F content .
- Critical Parameter Thresholds : Establish thresholds for film thickness (<10 nm) and bubble size (<100 µm) to predict foam collapse .
Q. How can computational models enhance the design of this compound-based materials?
- Molecular Dynamics (MD) : Simulate Al–F–Si network formation to predict glass transition temperatures and mechanical properties .
- Phase Diagram Construction : Use CALPHAD (CALculation of PHAse Diagrams) methods to map stability regions for Al–F–Si systems .
Q. Data Analysis and Interpretation
Q. What statistical approaches are suitable for analyzing variability in this compound synthesis outcomes?
- Design of Experiments (DoE) : Apply factorial designs to identify interactions between pH, temperature, and precursor ratios .
- Multivariate Regression : Correlate synthesis parameters (e.g., aging time) with properties like crystallinity or ion release rates .
Q. How can researchers reconcile discrepancies between theoretical predictions and experimental results in Al–F–Si systems?
- Error Source Identification : Quantify uncertainties in DFT calculations (e.g., basis set limitations) and experimental measurements (e.g., instrumental drift) .
- Sensitivity Analysis : Rank parameters (e.g., Al/F ratio) by their impact on model-experiment alignment .
Q. Future Research Directions
Q. What unresolved questions warrant further investigation in this compound research?
- Long-Term Environmental Impact : Assess Al/F leaching in natural waters using accelerated aging tests coupled with ecotoxicity assays .
- Advanced Characterization : Develop in-situ NMR techniques to monitor real-time structural evolution during synthesis .
- Multi-Scale Modeling : Bridge atomic-scale simulations with mesoscale foam stability models to predict macroscopic material behavior .
特性
CAS番号 |
17099-70-6 |
---|---|
分子式 |
Al2F18Si3 |
分子量 |
480.19 g/mol |
IUPAC名 |
dialuminum;hexafluorosilicon(2-) |
InChI |
InChI=1S/2Al.3F6Si/c;;3*1-7(2,3,4,5)6/q2*+3;3*-2 |
InChIキー |
YOKYIYGYAGPUHG-UHFFFAOYSA-N |
SMILES |
[F-].[F-].[F-].[F-].[F-].[F-].[F-].[F-].[F-].[F-].[F-].[F-].[F-].[F-].[F-].[F-].[F-].[F-].[Al+3].[Al+3].[Si+4].[Si+4].[Si+4] |
正規SMILES |
F[Si-2](F)(F)(F)(F)F.F[Si-2](F)(F)(F)(F)F.F[Si-2](F)(F)(F)(F)F.[Al+3].[Al+3] |
物理的記述 |
Nonahydrate: Solid; Soluble in water; [Merck Index] |
同義語 |
fluoroaluminum silicate |
製品の起源 |
United States |
試験管内研究製品の免責事項と情報
BenchChemで提示されるすべての記事および製品情報は、情報提供を目的としています。BenchChemで購入可能な製品は、生体外研究のために特別に設計されています。生体外研究は、ラテン語の "in glass" に由来し、生物体の外で行われる実験を指します。これらの製品は医薬品または薬として分類されておらず、FDAから任何の医療状態、病気、または疾患の予防、治療、または治癒のために承認されていません。これらの製品を人間または動物に体内に導入する形態は、法律により厳格に禁止されています。これらのガイドラインに従うことは、研究と実験において法的および倫理的な基準の遵守を確実にするために重要です。