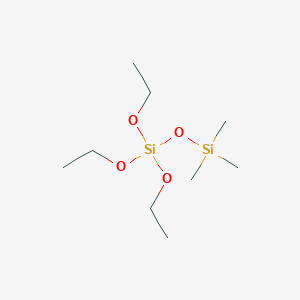
トリメチルシロキシトリエトキシシラン
- 専門家チームからの見積もりを受け取るには、QUICK INQUIRYをクリックしてください。
- 品質商品を競争力のある価格で提供し、研究に集中できます。
説明
Trimethylsiloxytriethoxysilane is an organosilicon compound with the chemical formula C9H24O4Si2. It is a colorless liquid known for its low boiling point and melting point. This compound is widely used in various industrial and scientific applications due to its unique chemical properties.
科学的研究の応用
Trimethylsiloxytriethoxysilane has a wide range of applications in scientific research, including:
Chemistry: It is used as a crosslinking agent and surface modifier in the synthesis of silicone-based materials.
Biology: The compound is used in the preparation of biocompatible materials for medical applications, such as drug delivery systems and tissue engineering scaffolds.
Medicine: Trimethylsiloxytriethoxysilane is utilized in the development of medical devices and implants due to its biocompatibility and stability.
作用機序
Target of Action
Trimethylsiloxytriethoxysilane is primarily used as a precursor in the synthesis of silicone materials Instead, its role is more of a chemical reactant in the production of various silicone-based materials .
Mode of Action
Trimethylsiloxytriethoxysilane contains both hydrolyzable siloxane bonds and an active silicon-hydrogen bond . These properties allow it to participate in a series of chemical reactions, such as copolymerization, polycondensation, and disproportionation reactions . The resulting changes depend on the specific reactions and the other reactants involved.
Pharmacokinetics
Pharmacokinetics typically refers to the absorption, distribution, metabolism, and excretion (ADME) of drugs within the bodyIn terms of chemical properties, it’s a clear, colorless liquid with a density of 096 g/mL .
Action Environment
The action, efficacy, and stability of Trimethylsiloxytriethoxysilane can be influenced by various environmental factors. For instance, the presence of moisture can lead to hydrolysis of the siloxane bonds . Additionally, the reaction conditions, such as temperature and pH, can also affect the outcome of the reactions it participates in .
準備方法
Synthetic Routes and Reaction Conditions: Trimethylsiloxytriethoxysilane is typically synthesized by reacting trichloromethylsilane with triethoxysilane under alkaline conditions . The reaction involves the substitution of chlorine atoms with ethoxy groups, resulting in the formation of trimethylsiloxytriethoxysilane.
Industrial Production Methods: In industrial settings, the synthesis of trimethylsiloxytriethoxysilane involves the use of a fixed-bed reactor. The reaction is catalyzed by copper chloride (CuCl) and metallic copper nanoparticles. The process is carried out at elevated temperatures, typically between 260°C and 500°C, to ensure the complete conversion of reactants to the desired product .
化学反応の分析
Types of Reactions: Trimethylsiloxytriethoxysilane undergoes various chemical reactions, including hydrolysis, condensation, and polymerization. These reactions are facilitated by the presence of hydrolyzable siloxane bonds and active silicon-hydrogen bonds.
Common Reagents and Conditions:
Hydrolysis: This reaction involves the cleavage of siloxane bonds in the presence of water, leading to the formation of silanols and ethanol.
Condensation: Silanols formed during hydrolysis can further condense to form siloxane bonds, resulting in the formation of polysiloxanes.
Polymerization: Trimethylsiloxytriethoxysilane can undergo polymerization reactions to form silicone-based polymers, which are used in various industrial applications.
Major Products Formed: The major products formed from these reactions include silanols, polysiloxanes, and silicone-based polymers. These products are utilized in the production of coatings, adhesives, and sealants.
類似化合物との比較
Trimethoxysilane: An organosilicon compound with similar hydrolyzable siloxane bonds and active silicon-hydrogen bonds.
Triethoxysilane: Another organosilicon compound used in surface modification and polymerization reactions.
Uniqueness of Trimethylsiloxytriethoxysilane: Trimethylsiloxytriethoxysilane is unique due to its combination of trimethylsiloxy and triethoxy groups, which provide it with distinct chemical properties. Its ability to form strong bonds with various substrates and its versatility in different chemical reactions make it a valuable compound in both scientific research and industrial applications .
特性
IUPAC Name |
triethyl trimethylsilyl silicate |
Source
|
---|---|---|
Source | PubChem | |
URL | https://pubchem.ncbi.nlm.nih.gov | |
Description | Data deposited in or computed by PubChem | |
InChI |
InChI=1S/C9H24O4Si2/c1-7-10-15(11-8-2,12-9-3)13-14(4,5)6/h7-9H2,1-6H3 |
Source
|
Source | PubChem | |
URL | https://pubchem.ncbi.nlm.nih.gov | |
Description | Data deposited in or computed by PubChem | |
InChI Key |
OPVRXFLDMSPNDD-UHFFFAOYSA-N |
Source
|
Source | PubChem | |
URL | https://pubchem.ncbi.nlm.nih.gov | |
Description | Data deposited in or computed by PubChem | |
Canonical SMILES |
CCO[Si](OCC)(OCC)O[Si](C)(C)C |
Source
|
Source | PubChem | |
URL | https://pubchem.ncbi.nlm.nih.gov | |
Description | Data deposited in or computed by PubChem | |
Molecular Formula |
C9H24O4Si2 |
Source
|
Source | PubChem | |
URL | https://pubchem.ncbi.nlm.nih.gov | |
Description | Data deposited in or computed by PubChem | |
DSSTOX Substance ID |
DTXSID90540420 |
Source
|
Record name | Triethyl trimethylsilyl orthosilicate | |
Source | EPA DSSTox | |
URL | https://comptox.epa.gov/dashboard/DTXSID90540420 | |
Description | DSSTox provides a high quality public chemistry resource for supporting improved predictive toxicology. | |
Molecular Weight |
252.45 g/mol |
Source
|
Source | PubChem | |
URL | https://pubchem.ncbi.nlm.nih.gov | |
Description | Data deposited in or computed by PubChem | |
CAS No. |
17861-35-7 |
Source
|
Record name | Triethyl trimethylsilyl orthosilicate | |
Source | EPA DSSTox | |
URL | https://comptox.epa.gov/dashboard/DTXSID90540420 | |
Description | DSSTox provides a high quality public chemistry resource for supporting improved predictive toxicology. | |
試験管内研究製品の免責事項と情報
BenchChemで提示されるすべての記事および製品情報は、情報提供を目的としています。BenchChemで購入可能な製品は、生体外研究のために特別に設計されています。生体外研究は、ラテン語の "in glass" に由来し、生物体の外で行われる実験を指します。これらの製品は医薬品または薬として分類されておらず、FDAから任何の医療状態、病気、または疾患の予防、治療、または治癒のために承認されていません。これらの製品を人間または動物に体内に導入する形態は、法律により厳格に禁止されています。これらのガイドラインに従うことは、研究と実験において法的および倫理的な基準の遵守を確実にするために重要です。