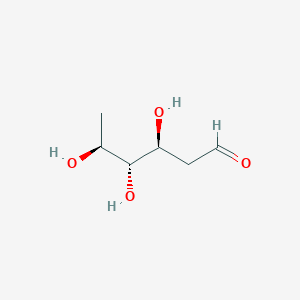
2-Deoxy-L-fucose
概要
説明
2-Deoxy-2-fluoro-L-fucose is an L-fucose analog and a fucosylation inhibitor . It inhibits the de novo synthesis of GDP-fucose in mammalian cells . Fucosylation is a well-defined biomarker for progression in many human cancers, such as pancreatic and hepatocellular carcinoma .
Synthesis Analysis
The synthesis of 2-Deoxy-2-fluoro-L-fucose involves the inhibition of de novo synthesis of GDP-fucose in mammalian cells . It’s also reported that 2-Deoxy-2-fluoro-L-fucose inhibits the efficient production of short-chain fatty acids and is involved in cross-feeding microbial interactions .Molecular Structure Analysis
The molecular formula of 2-Deoxy-2-fluoro-L-fucose is C6H11FO4 . Its average mass is 166.148 Da and its monoisotopic mass is 166.064133 Da .Chemical Reactions Analysis
2-Deoxy-2-fluoro-L-fucose is a potent inhibitor of the plant cell elongation . After feeding plant seedlings with 2F-Fuc, this monosaccharide derivative is deacetylated and converted by the endogenous metabolic machinery into the corresponding nucleotide-sugar, which then efficiently inhibits Golgi-localized fucosyltransferases .Physical And Chemical Properties Analysis
2-Deoxy-2-fluoro-L-fucose has a molecular formula of C6H11FO4 . Its average mass is 166.148 Da and its monoisotopic mass is 166.064133 Da .科学的研究の応用
Overview
L-Fucose, a natural deoxy hexose, has a variety of physiological effects and potential applications in the pharmaceutical, cosmetic, and food industries. Microbial synthesis via metabolic engineering is an efficient way to produce L-Fucose. Notably, a metabolically engineered Escherichia coli strain demonstrated high L-Fucose productivity with titers reaching up to 51.05 g/L in fed-batch cultivation. The synthesis was not significantly affected by lactose, and there was minimal residue of 2'-fucosyllactose throughout the cultivation processes, showing significant potential for industrial applications (Meng et al., 2023).
Biological Functions of Fucose in Mammals
Overview
Fucose is involved in various biological functions in mammals, including immunity and cancer. Fucose modifications have been implicated in developmental processes in mice and human disorders like leukocyte adhesion deficiency type II. The altered levels of fucosylated proteins serve as diagnostic tools for cancer. Furthermore, chemically modified fucose analogs can be used to alter fucose-dependent processes or as tools for understanding them (Schneider et al., 2017).
Engineering Escherichia coli for L-Fucose Production
Overview
The production of L-Fucose can be achieved by engineering Escherichia coli. Modifications to the strain genome allowed for efficient production of L-Fucose from 2′-fucosyllactose (2′-FL), demonstrating an efficient one-pot biosynthesis strategy. This makes large-scale industrial production of L-Fucose feasible, with a production of 16.7 g/L of L-Fucose in a fed-batch fermentation (Liu et al., 2019).
Fucose: Biosynthesis and Biological Function in Mammals
Overview
Fucose-containing glycans in mammals play crucial roles in various biological processes, including blood transfusion reactions, leukocyte-endothelial adhesion, and signaling by the Notch receptor family. Alterations in fucosylated oligosaccharides are observed in pathological processes like cancer and atherosclerosis. The deficiency of fucose leads to complex phenotypes in humans and mice, emphasizing the importance of fucosylated glycans and the biosynthetic processes leading to the formation of GDP-fucose (Becker & Lowe, 2003).
作用機序
Safety and Hazards
将来の方向性
A bacterial biosensor of fucose was validated with good sensitivity and precision . A biological method for quantifying fucose could be useful for nutraceutical and microbiological applications, as well as molecular diagnostics . The light-controlled release of 2-fluoro-l-fucose, an inhibitor of the root cell elongation, from a nitrobenzyl-caged derivative has also been studied .
特性
IUPAC Name |
(3S,4R,5S)-3,4,5-trihydroxyhexanal | |
---|---|---|
Source | PubChem | |
URL | https://pubchem.ncbi.nlm.nih.gov | |
Description | Data deposited in or computed by PubChem | |
InChI |
InChI=1S/C6H12O4/c1-4(8)6(10)5(9)2-3-7/h3-6,8-10H,2H2,1H3/t4-,5-,6+/m0/s1 | |
Source | PubChem | |
URL | https://pubchem.ncbi.nlm.nih.gov | |
Description | Data deposited in or computed by PubChem | |
InChI Key |
JWFRNGYBHLBCMB-HCWXCVPCSA-N | |
Source | PubChem | |
URL | https://pubchem.ncbi.nlm.nih.gov | |
Description | Data deposited in or computed by PubChem | |
Canonical SMILES |
CC(C(C(CC=O)O)O)O | |
Source | PubChem | |
URL | https://pubchem.ncbi.nlm.nih.gov | |
Description | Data deposited in or computed by PubChem | |
Isomeric SMILES |
C[C@@H]([C@H]([C@H](CC=O)O)O)O | |
Source | PubChem | |
URL | https://pubchem.ncbi.nlm.nih.gov | |
Description | Data deposited in or computed by PubChem | |
Molecular Formula |
C6H12O4 | |
Source | PubChem | |
URL | https://pubchem.ncbi.nlm.nih.gov | |
Description | Data deposited in or computed by PubChem | |
DSSTOX Substance ID |
DTXSID30940809 | |
Record name | 2,6-Dideoxyhexose | |
Source | EPA DSSTox | |
URL | https://comptox.epa.gov/dashboard/DTXSID30940809 | |
Description | DSSTox provides a high quality public chemistry resource for supporting improved predictive toxicology. | |
Molecular Weight |
148.16 g/mol | |
Source | PubChem | |
URL | https://pubchem.ncbi.nlm.nih.gov | |
Description | Data deposited in or computed by PubChem | |
Product Name |
2-Deoxy-L-fucose | |
CAS RN |
19165-06-1 | |
Record name | 2-Deoxy-L-fucose | |
Source | ChemIDplus | |
URL | https://pubchem.ncbi.nlm.nih.gov/substance/?source=chemidplus&sourceid=0019165061 | |
Description | ChemIDplus is a free, web search system that provides access to the structure and nomenclature authority files used for the identification of chemical substances cited in National Library of Medicine (NLM) databases, including the TOXNET system. | |
Record name | 2,6-Dideoxyhexose | |
Source | EPA DSSTox | |
URL | https://comptox.epa.gov/dashboard/DTXSID30940809 | |
Description | DSSTox provides a high quality public chemistry resource for supporting improved predictive toxicology. | |
Retrosynthesis Analysis
AI-Powered Synthesis Planning: Our tool employs the Template_relevance Pistachio, Template_relevance Bkms_metabolic, Template_relevance Pistachio_ringbreaker, Template_relevance Reaxys, Template_relevance Reaxys_biocatalysis model, leveraging a vast database of chemical reactions to predict feasible synthetic routes.
One-Step Synthesis Focus: Specifically designed for one-step synthesis, it provides concise and direct routes for your target compounds, streamlining the synthesis process.
Accurate Predictions: Utilizing the extensive PISTACHIO, BKMS_METABOLIC, PISTACHIO_RINGBREAKER, REAXYS, REAXYS_BIOCATALYSIS database, our tool offers high-accuracy predictions, reflecting the latest in chemical research and data.
Strategy Settings
Precursor scoring | Relevance Heuristic |
---|---|
Min. plausibility | 0.01 |
Model | Template_relevance |
Template Set | Pistachio/Bkms_metabolic/Pistachio_ringbreaker/Reaxys/Reaxys_biocatalysis |
Top-N result to add to graph | 6 |
Feasible Synthetic Routes
試験管内研究製品の免責事項と情報
BenchChemで提示されるすべての記事および製品情報は、情報提供を目的としています。BenchChemで購入可能な製品は、生体外研究のために特別に設計されています。生体外研究は、ラテン語の "in glass" に由来し、生物体の外で行われる実験を指します。これらの製品は医薬品または薬として分類されておらず、FDAから任何の医療状態、病気、または疾患の予防、治療、または治癒のために承認されていません。これらの製品を人間または動物に体内に導入する形態は、法律により厳格に禁止されています。これらのガイドラインに従うことは、研究と実験において法的および倫理的な基準の遵守を確実にするために重要です。