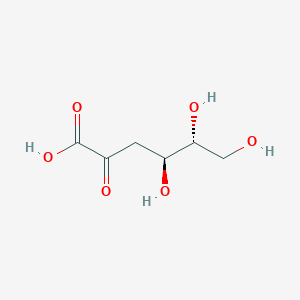
2-ケト-3-デオキシグルコン酸
説明
2-Keto-3-deoxygluconate (2-KDG) is a sugar molecule that is found naturally in the body and is important for a variety of biochemical and physiological functions. It is a key intermediate in the pentose phosphate pathway, which is responsible for the production of NADPH, a molecule that is essential for the biosynthesis of fatty acids, cholesterol, and other important molecules. 2-KDG is also involved in the regulation of gene expression, and it has been shown to have anti-inflammatory, anti-oxidant, and anti-cancer properties.
科学的研究の応用
代謝経路研究
KDGは、糖の分解のためのエントナー・ドゥドロフ経路、糖酸や糖ポリマーの分解など、様々な代謝経路において重要な役割を果たしています . KDGの代謝を研究することで、これらの経路の調節と機能に関する洞察を得ることができます.
バイオテクノロジー的用途
バイオテクノロジーなど、多くの分野では、KDGのような代謝中間体の利用が必須です . 例えば、KDGは、エントナー・ドゥドロフ経路の重要な中間体である、2-ケト-3-デオキシ-6-ホスホグルコン酸(KDPG)の酵素合成に使用できます .
生物医学的用途
生物医学分野では、KDGは代謝中間体としての役割を果たすため、使用することができます . 例えば、エントナー・ドゥドロフ経路を利用するヒトや植物の病原体の研究に使用できます .
診断的用途
KDGは、様々な代謝経路における役割から診断に使用することができます . これらの経路の研究は、様々な病気や状態の診断に役立ちます。
酵素合成
KDGは、他の化合物の酵素合成に使用できます。 例えば、KDPGの酵素合成に使用されています .
アミノ酸の生産
KDGはアミノ酸の生産に使用できます。 例えば、KDGからL-アラニンとL-セリンを同時に合成する、マルチ酵素反応カスケードが開発されています .
ペクチンの分解
エルウィニア・クリサンセミでは、KDGはペクチン分解に関与するkdgレギュロンのすべての遺伝子の発現を可能にする、KdgRと相互作用する誘導分子です .
作用機序
2-Keto-3-deoxygluconate (KDG)
is a key intermediate in the Entner-Doudoroff (ED) pathway , a significant route for sugar and sugar acid degradation in many organisms . Here is an overview of its mechanism of action:
Target of Action
The primary target of 2-Keto-3-deoxygluconate is the enzyme 2-keto-3-deoxy-gluconate kinase , found in organisms such as Thermus thermophilus . This enzyme plays a crucial role in the phosphorylation process of the ED pathway .
Mode of Action
2-Keto-3-deoxygluconate interacts with its target enzyme, 2-keto-3-deoxy-gluconate kinase, in a process that involves the transfer of a phosphate group from ATP to 2-Keto-3-deoxygluconate . This interaction results in the formation of 6-phospho-2-dehydro-3-deoxy-D-gluconate .
Biochemical Pathways
The ED pathway, in which 2-Keto-3-deoxygluconate plays a key role, is utilized by a significant proportion of heterotrophic prokaryotic microorganisms for sugar and sugar acid degradation . This pathway also plays a role in the degradation of pectin and alginate, polymers used for biofuel production and generation of high-value-added products .
Pharmacokinetics
It is known that the compound is involved in the ed pathway, which operates under aerobic conditions and is stimulated by phosphate starvation .
Result of Action
The action of 2-Keto-3-deoxygluconate results in the production of key metabolic intermediates, providing ATP, NAD(P)H, and biosynthetic precursors for amino acids, nucleotides, and fatty acids . It also contributes to the degradation of pectin and alginate, serving as a carbon and energy source in bacterial cells .
Action Environment
The action of 2-Keto-3-deoxygluconate is influenced by environmental factors such as light, glucose availability, and phosphate levels . The ED pathway, in which this compound is involved, is particularly significant under mixotrophic conditions (light and glucose) and under autotrophic conditions in a day/night cycle .
将来の方向性
Enzymatic reaction cascades represent a powerful tool to convert biogenic resources into valuable chemicals for fuel and commodity markets . KDG, as a sugar degradation product, can be used as a precursor in these biocatalytic conversion strategies . For instance, a novel, multi-enzymatic reaction cascade for the one-pot simultaneous synthesis of L-alanine and L-serine from KDG has been described .
生化学分析
Biochemical Properties
2-Keto-3-deoxygluconate interacts with several enzymes and proteins. One of the key enzymes it interacts with is 2-dehydro-3-deoxygluconokinase . This enzyme catalyzes the chemical reaction involving ATP and 2-dehydro-3-deoxy-D-gluconate, producing ADP and 6-phospho-2-dehydro-3-deoxy-D-gluconate . Another enzyme, 6-Phosphogluconate-Dehydratase, is involved in the enzymatic dehydration of 6-phosphogluconate to produce 2-Keto-3-deoxy-6-phosphogluconate .
Cellular Effects
The presence of 2-Keto-3-deoxygluconate in a cell can influence various cellular processes. It is a key metabolite in the ED pathway, which is integral to the catabolism of sugars and sugar acids . This pathway is crucial for the energy metabolism of many microorganisms . The compound’s influence on cell function includes its impact on metabolic flux, as it is a key intermediate in these metabolic pathways .
Molecular Mechanism
The molecular mechanism of 2-Keto-3-deoxygluconate involves its interaction with various enzymes. For instance, 2-dehydro-3-deoxygluconokinase catalyzes a reaction that transforms ATP and 2-dehydro-3-deoxy-D-gluconate into ADP and 6-phospho-2-dehydro-3-deoxy-D-gluconate . This reaction is part of the transfer of phosphorus-containing groups, with an alcohol group as the acceptor .
Temporal Effects in Laboratory Settings
It is known that this compound is a key intermediate in the degradation of sugars and sugar acids, and its presence is crucial for the functioning of the ED pathway .
Metabolic Pathways
2-Keto-3-deoxygluconate is involved in the ED pathway, a key metabolic pathway for the degradation of sugars and sugar acids . This pathway is utilized by a significant proportion of heterotrophic prokaryotic microorganisms . The compound is also a product of the degradation of pectin and alginate, two polysaccharides that are primary-based compounds for biofuel production and for the generation of high-value-added products .
Transport and Distribution
The transport and distribution of 2-Keto-3-deoxygluconate within cells and tissues involve specific transporters. For instance, the 2-Keto-3-deoxygluconate transporter from Bacillus subtilis and Erwinia chrysanthemi is known to facilitate the uptake of this compound into the cell .
特性
IUPAC Name |
(4R,5R)-4,5,6-trihydroxy-2-oxohexanoic acid | |
---|---|---|
Source | PubChem | |
URL | https://pubchem.ncbi.nlm.nih.gov | |
Description | Data deposited in or computed by PubChem | |
InChI |
InChI=1S/C6H10O6/c7-2-5(10)3(8)1-4(9)6(11)12/h3,5,7-8,10H,1-2H2,(H,11,12)/t3-,5-/m1/s1 | |
Source | PubChem | |
URL | https://pubchem.ncbi.nlm.nih.gov | |
Description | Data deposited in or computed by PubChem | |
InChI Key |
WPAMZTWLKIDIOP-NQXXGFSBSA-N | |
Source | PubChem | |
URL | https://pubchem.ncbi.nlm.nih.gov | |
Description | Data deposited in or computed by PubChem | |
Canonical SMILES |
C(C(C(CO)O)O)C(=O)C(=O)O | |
Source | PubChem | |
URL | https://pubchem.ncbi.nlm.nih.gov | |
Description | Data deposited in or computed by PubChem | |
Isomeric SMILES |
C([C@H]([C@@H](CO)O)O)C(=O)C(=O)O | |
Source | PubChem | |
URL | https://pubchem.ncbi.nlm.nih.gov | |
Description | Data deposited in or computed by PubChem | |
Molecular Formula |
C6H10O6 | |
Source | PubChem | |
URL | https://pubchem.ncbi.nlm.nih.gov | |
Description | Data deposited in or computed by PubChem | |
DSSTOX Substance ID |
DTXSID201344318 | |
Record name | 2-Oxo-3-deoxygalactonic acid | |
Source | EPA DSSTox | |
URL | https://comptox.epa.gov/dashboard/DTXSID201344318 | |
Description | DSSTox provides a high quality public chemistry resource for supporting improved predictive toxicology. | |
Molecular Weight |
178.14 g/mol | |
Source | PubChem | |
URL | https://pubchem.ncbi.nlm.nih.gov | |
Description | Data deposited in or computed by PubChem | |
Physical Description |
Solid | |
Record name | 2-Keto-3-deoxy-D-gluconic acid | |
Source | Human Metabolome Database (HMDB) | |
URL | http://www.hmdb.ca/metabolites/HMDB0001353 | |
Description | The Human Metabolome Database (HMDB) is a freely available electronic database containing detailed information about small molecule metabolites found in the human body. | |
Explanation | HMDB is offered to the public as a freely available resource. Use and re-distribution of the data, in whole or in part, for commercial purposes requires explicit permission of the authors and explicit acknowledgment of the source material (HMDB) and the original publication (see the HMDB citing page). We ask that users who download significant portions of the database cite the HMDB paper in any resulting publications. | |
CAS No. |
56742-44-0, 17510-99-5 | |
Record name | 2-Oxo-3-deoxygalactonic acid | |
Source | ChemIDplus | |
URL | https://pubchem.ncbi.nlm.nih.gov/substance/?source=chemidplus&sourceid=0056742440 | |
Description | ChemIDplus is a free, web search system that provides access to the structure and nomenclature authority files used for the identification of chemical substances cited in National Library of Medicine (NLM) databases, including the TOXNET system. | |
Record name | 2-Oxo-3-deoxygalactonic acid | |
Source | EPA DSSTox | |
URL | https://comptox.epa.gov/dashboard/DTXSID201344318 | |
Description | DSSTox provides a high quality public chemistry resource for supporting improved predictive toxicology. | |
Record name | 2-Keto-3-deoxy-D-gluconic acid | |
Source | Human Metabolome Database (HMDB) | |
URL | http://www.hmdb.ca/metabolites/HMDB0001353 | |
Description | The Human Metabolome Database (HMDB) is a freely available electronic database containing detailed information about small molecule metabolites found in the human body. | |
Explanation | HMDB is offered to the public as a freely available resource. Use and re-distribution of the data, in whole or in part, for commercial purposes requires explicit permission of the authors and explicit acknowledgment of the source material (HMDB) and the original publication (see the HMDB citing page). We ask that users who download significant portions of the database cite the HMDB paper in any resulting publications. | |
Retrosynthesis Analysis
AI-Powered Synthesis Planning: Our tool employs the Template_relevance Pistachio, Template_relevance Bkms_metabolic, Template_relevance Pistachio_ringbreaker, Template_relevance Reaxys, Template_relevance Reaxys_biocatalysis model, leveraging a vast database of chemical reactions to predict feasible synthetic routes.
One-Step Synthesis Focus: Specifically designed for one-step synthesis, it provides concise and direct routes for your target compounds, streamlining the synthesis process.
Accurate Predictions: Utilizing the extensive PISTACHIO, BKMS_METABOLIC, PISTACHIO_RINGBREAKER, REAXYS, REAXYS_BIOCATALYSIS database, our tool offers high-accuracy predictions, reflecting the latest in chemical research and data.
Strategy Settings
Precursor scoring | Relevance Heuristic |
---|---|
Min. plausibility | 0.01 |
Model | Template_relevance |
Template Set | Pistachio/Bkms_metabolic/Pistachio_ringbreaker/Reaxys/Reaxys_biocatalysis |
Top-N result to add to graph | 6 |
Feasible Synthetic Routes
Q1: What is 2-keto-3-deoxygluconate (KDG) and what is its role in bacterial metabolism?
A: 2-keto-3-deoxygluconate (KDG) is a key intermediate in the bacterial catabolism of hexuronates, such as galacturonate and glucuronate, derived from pectin and alginate. [, ] These polysaccharides are major components of plant cell walls and represent an important carbon source for many bacteria. [] KDG is further metabolized to pyruvate, a central metabolite that can enter various metabolic pathways. [, , , ]
Q2: How is KDG produced in bacteria?
A: KDG is produced from either galacturonate or glucuronate through separate three-step pathways. [] These pathways converge at the formation of KDG, which is further metabolized by the enzymes KDG kinase (KDGK) and KDG aldolase (KDGA). [, , ]
Q3: How is KDG transport regulated in bacteria?
A: KDG uptake is mediated by a specific transport system encoded by the kdgT gene. [, ] The expression of kdgT, along with other genes involved in pectin degradation, is negatively regulated by the kdgR gene. [, , , ] KDG itself acts as an inducer, binding to KdgR and relieving the repression. [, , , ]
Q4: How does iron availability affect KDG metabolism and pectin degradation in bacteria like Erwinia chrysanthemi?
A: In E. chrysanthemi, iron limitation activates the transcription of genes involved in both iron transport and pectin degradation, including those responsible for KDG metabolism. [] This coregulation is mediated by the ferric uptake regulator (Fur) protein, which senses iron availability. [] KDG itself can also induce the expression of iron transport genes, suggesting a complex interplay between iron assimilation and pectinolysis in this bacterium. []
Q5: What is the role of 2-keto-3-deoxygluconate aldolase (KDGA) in KDG metabolism?
A: KDGA catalyzes the reversible aldol cleavage of KDG into pyruvate and glyceraldehyde. [, , , ] This reaction is a crucial step in the non-phosphorylative Entner-Doudoroff pathway utilized by some bacteria and archaea for glucose metabolism. [, , , , , , ]
Q6: Is KDGA specific to KDG?
A: While some KDGAs show high specificity for KDG, others exhibit catalytic promiscuity. For instance, KDGA from Sulfolobus solfataricus can also cleave 2-keto-3-deoxygalactonate (KDGal) with comparable efficiency. [, , ] This promiscuity allows S. solfataricus to utilize both glucose and galactose through a single, non-phosphorylative Entner-Doudoroff pathway. [, , ]
Q7: How does the structure of KDGA contribute to its substrate specificity and promiscuity?
A: Structural studies on KDGAs from different organisms have provided insights into the basis of their substrate specificity and promiscuity. [, , , ] For example, the crystal structure of KDGA from S. solfataricus revealed a spacious active site that can accommodate both KDG and KDGal. [, ] This observation explains the enzyme's ability to cleave both substrates. [, ] In contrast, KDGA from Picrophilus torridus has a more restricted active site that favors KDG binding. []
Q8: Can the stereoselectivity of KDGA be engineered?
A: Yes, site-directed mutagenesis based on structural information has been successfully employed to alter the stereoselectivity of KDGA. [, ] For example, researchers have generated mutant variants of KDGA from S. solfataricus that can selectively produce either D-KDG or D-KDGal from pyruvate and D-glyceraldehyde. [] These engineered enzymes have potential applications in the synthesis of rare sugars and other valuable compounds. []
Q9: Do all bacteria utilize the same pathway for KDG metabolism?
A: No, variations in the KDG metabolic pathway exist among bacteria. While some utilize a non-phosphorylative pathway, others employ a semi-phosphorylative route. [] For example, Sulfolobus solfataricus primarily relies on a non-phosphorylative pathway, but evidence suggests the presence of a parallel semi-phosphorylative pathway involving a KDG kinase. [, ]
Q10: How does the KDG pathway in archaea differ from that in bacteria?
A: Archaea like Sulfolobus solfataricus and Picrophilus torridus utilize a non-phosphorylative Entner-Doudoroff pathway involving a KDG-specific aldolase. [, ] This pathway differs from the semi-phosphorylative pathway found in some bacteria, where KDG is phosphorylated to 2-keto-3-deoxy-6-phosphogluconate (KDPG) before aldol cleavage. [, ]
Q11: What is the evolutionary significance of the KDG pathway?
A: The presence of the KDG pathway in both bacteria and archaea suggests an ancient origin for this metabolic route. [] The variations observed in different organisms highlight the evolutionary adaptation of this pathway to specific ecological niches and metabolic requirements. [, ]
Q12: What are the potential biotechnological applications of KDG and its metabolizing enzymes?
A: KDG and its metabolizing enzymes, particularly KDGA, hold potential for various biotechnological applications. [] Engineered KDGAs could be used to synthesize rare sugars, such as L-KDG and L-KDGal, which are challenging to obtain chemically. [] These sugars have potential applications as building blocks for pharmaceuticals and other valuable compounds. [] Furthermore, understanding the regulation of KDG metabolism in plant-pathogenic bacteria could lead to novel strategies for controlling plant diseases. []
試験管内研究製品の免責事項と情報
BenchChemで提示されるすべての記事および製品情報は、情報提供を目的としています。BenchChemで購入可能な製品は、生体外研究のために特別に設計されています。生体外研究は、ラテン語の "in glass" に由来し、生物体の外で行われる実験を指します。これらの製品は医薬品または薬として分類されておらず、FDAから任何の医療状態、病気、または疾患の予防、治療、または治癒のために承認されていません。これらの製品を人間または動物に体内に導入する形態は、法律により厳格に禁止されています。これらのガイドラインに従うことは、研究と実験において法的および倫理的な基準の遵守を確実にするために重要です。