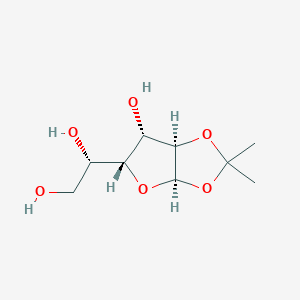
1,2-O-Isopropylidene-alpha-D-glucofuranose
概要
説明
1,2-O-Isopropylidene-α-D-glucofuranose is a protected carbohydrate derivative widely used in organic synthesis and pharmaceutical research. Its structure features a glucofuranose core with a 1,2-O-isopropylidene acetal group, which stabilizes the furanose ring and directs regioselective modifications. The compound is synthesized from D-glucose via acetonation under acidic conditions, achieving high yields (91–98%) using acetone and sulfuric acid . Key applications include its role as a precursor for glycosylation reactions, antiviral drug intermediates, and glucose metabolism therapies . Its molecular formula is C₉H₁₆O₆ (MW: 220.22), with CAS No. 18549-40-1 .
準備方法
Synthesis Methods
Acid-Catalyzed Acetonation of D-Glucose
The most common method for synthesizing IpGlc involves the reaction of D-glucose with acetone in the presence of an acid catalyst. This process selectively protects the 1,2-hydroxyl groups, favoring the furanose form due to kinetic control.
Standard Protocol
-
Reagents : D-glucose (1 equiv), acetone (excess), acid catalyst (e.g., HCl, H2SO4, or p-toluenesulfonic acid).
-
Procedure :
-
D-glucose is suspended in acetone, and the acid catalyst is added (e.g., 0.1 M HCl) .
-
The mixture is heated at 80°C for 1 hour under reflux to drive acetal formation .
-
The reaction is neutralized (e.g., with NaHCO3), and acetone is evaporated under reduced pressure.
-
The residue is purified via recrystallization from hexane or ether/hexane mixtures .
-
Yield : ~70–85% under optimized conditions .
Catalytic Variations
-
HCl vs. H2SO4 : Hydrochloric acid (0.1 M) is preferred for milder conditions, minimizing side products like di-O-isopropylidene derivatives . Concentrated H2SO4 may increase reaction rates but risks over-acidification and decomposition.
-
Solvent Systems : Anhydrous acetone ensures high solubility of D-glucose, though mixtures with DCM or pyridine are used in derivatization steps .
Large-Scale Synthesis and Optimization
For industrial applications, scalability and cost-efficiency are paramount. Key parameters include:
Temperature and Time
-
Optimal Range : 50–80°C . At 80°C, reactions complete within 1 hour, whereas lower temperatures (50°C) require extended periods (24–72 hours) .
-
Trade-offs : Higher temperatures accelerate kinetics but may degrade acid-sensitive products.
Substrate Concentration
-
IpGlc Solubility : At 40°C, solubility in acetone is ~200 mmol/kg, enabling high substrate loading without precipitation .
-
Effect on Yield : Initial IpGlc concentrations ≤200 mmol/L achieve ~45–55% conversion in lipase-catalyzed esterifications, while concentrations ≥400 mmol/L require longer durations (7 days) for comparable yields .
Purification and Characterization
Purification Techniques
-
Recrystallization :
-
Column Chromatography :
Spectroscopic Characterization
-
1H NMR (CDCl3) :
-
13C NMR :
Applications in Further Synthesis
Lipase-Catalyzed Esterification
IpGlc serves as a substrate for synthesizing 6-O-acyl derivatives (e.g., 6-O-octanoyl-IpGlc) using immobilized Candida antarctica lipase B (CALB) in acetone .
Conditions :
Outcome :
Tosylation and Azide Substitution
IpGlc is tosylated at the 6-position for nucleophilic displacement with azide, yielding 6-azido-6-deoxy derivatives .
Procedure :
Yield : 29% after recrystallization .
Physicochemical Properties
Solubility Profile
Solvent | Solubility (mmol/kg, 40°C) |
---|---|
Acetone | 200 |
Acetonitrile | 45 |
tert-Amyl alcohol | 30 |
Thermal Stability
-
Decomposition Temperature : >150°C (DSC).
-
Storage : Stable at −20°C under anhydrous conditions for >1 year .
Challenges and Mitigation Strategies
By-Product Formation
-
Di-O-isopropylidene Derivatives : Formed under prolonged heating or excess acetone. Mitigated by controlling reaction time and acid concentration .
-
Hydrolysis : Moisture exposure reverses acetal formation. Use anhydrous solvents and molecular sieves .
Enzyme Inhibition
High IpGlc concentrations (>200 mmol/L) inhibit lipase activity in esterification reactions. Solution: Semi-batch addition of substrate .
化学反応の分析
Types of Reactions: 1,2-O-Isopropylidene-alpha-D-glucofuranose undergoes various chemical reactions, including:
Oxidation: The compound can be oxidized to form corresponding carboxylic acids or aldehydes.
Reduction: Reduction reactions can convert the compound into alcohol derivatives.
Substitution: The hydroxyl groups can be substituted with other functional groups using appropriate reagents.
Common Reagents and Conditions:
Oxidation: Common oxidizing agents include potassium permanganate (KMnO4) and chromium trioxide (CrO3).
Reduction: Reducing agents such as sodium borohydride (NaBH4) or lithium aluminum hydride (LiAlH4) are used.
Substitution: Reagents like acetyl chloride (CH3COCl) or benzoyl chloride (C6H5COCl) can be used for substitution reactions.
Major Products:
Oxidation: Carboxylic acids or aldehydes.
Reduction: Alcohol derivatives.
Substitution: Ester or ether derivatives.
科学的研究の応用
Food Industry
- Sugar Substitute : This compound serves as a low-calorie sweetener alternative in food products, making it suitable for diabetic-friendly formulations. Its sweetness profile mimics that of traditional sugars without the associated caloric intake .
- Functional Ingredients : It can enhance the texture and mouthfeel of food products, contributing to improved consumer acceptance and satisfaction.
Pharmaceuticals
- Synthesis of Glycosides : 1,2-O-Isopropylidene-alpha-D-glucofuranose is utilized in synthesizing various glycosides, which are crucial for drug formulation. It enhances the stability and bioavailability of pharmaceutical compounds, thereby improving therapeutic efficacy .
- Antibacterial Properties : Recent studies have shown that derivatives of this compound exhibit antibacterial activity against several pathogens, making it a candidate for developing new antimicrobial agents .
Biotechnology
- Protecting Group in Organic Synthesis : In carbohydrate chemistry, this compound acts as a protecting group for hydroxyl functionalities during synthetic processes. This feature allows chemists to selectively modify other parts of the molecule without affecting the protected hydroxyl groups .
- Research Tool : It is frequently employed in academic research to explore carbohydrate-related biological processes and develop novel carbohydrate-based materials .
Cosmetics
- Moisturizing Agent : The compound is incorporated into skincare formulations due to its ability to enhance skin hydration and texture. Its use in cosmetics appeals to consumers looking for effective moisturizing solutions .
Case Study 1: Antibacterial Screening
A study conducted by Kawsar et al. (2013) explored the antibacterial properties of various derivatives of this compound against six human pathogenic bacteria. The results indicated that these derivatives exhibited moderate antibacterial activity, particularly against Gram-positive bacteria compared to Gram-negative strains. The study utilized FTIR and NMR spectroscopy for structural characterization of the synthesized compounds .
Case Study 2: Synthesis of Glycosides
In another research effort, this compound was used as a starting material for synthesizing biologically active compounds such as L-acovenose and carbanucleosides. The study demonstrated the efficiency of using this compound in creating complex carbohydrates with potential therapeutic applications .
Data Tables
Application Area | Specific Use Case | Key Findings |
---|---|---|
Food Industry | Sugar substitute | Low-calorie sweetener suitable for diabetic diets |
Pharmaceuticals | Synthesis of glycosides | Enhances drug stability and bioavailability |
Biotechnology | Protecting group in organic synthesis | Allows selective modification of carbohydrate structures |
Cosmetics | Moisturizing agent | Improves skin hydration and texture |
Antibacterial Research | Derivatives show moderate antibacterial activity | Effective against Gram-positive bacteria |
作用機序
The mechanism of action of 1,2-O-Isopropylidene-alpha-D-glucofuranose involves its role as a protecting group. The isopropylidene group protects the hydroxyl groups at positions 1 and 2 of the glucose molecule, preventing unwanted reactions during chemical synthesis . This protection is crucial in multi-step synthesis processes where selective reactions are required . The isopropylidene group can be easily removed under acidic conditions, restoring the original hydroxyl groups .
類似化合物との比較
Structural and Functional Modifications
Table 1: Key Derivatives and Their Properties
Reactivity and Selectivity
- Sulfonyl Derivatives : 6-O-sulfonyl groups (e.g., methylsulfonyl, tosyl) enhance leaving-group ability, facilitating nucleophilic substitution at C-4. These derivatives are pivotal in synthesizing glycosides and glycoconjugates .
- Acylated Derivatives: 6-O-acyl esters (e.g., octanoyl, lauroyl) exhibit antimicrobial activity, with higher efficacy against Gram-positive bacteria due to membrane disruption .
- Benzylidene and Sulfinyl Groups : 3,5-O-benzylidene derivatives enable stereochemical control in diastereomer synthesis, while sulfinyl groups at C-3 promote asymmetric reactions .
- Anhydro Derivatives : 5,6-Anhydro rings undergo cycloaddition with dienes, forming bicyclic structures for natural product synthesis .
Table 2: Antimicrobial Efficacy of Selected Derivatives
Compound | MIC (μg/mL) Against Gram-Positive Bacteria | MIC (μg/mL) Against Gram-Negative Bacteria |
---|---|---|
6-O-Lauroyl-α-D-glucofuranose | 12.5–25 | 50–100 |
6-O-Octanoyl-α-D-glucofuranose | 25–50 | 100–200 |
3,5,6-Tri-O-acetyl-α-D-glucofuranose | 50–100 | >200 |
MIC: Minimum Inhibitory Concentration
Acylated derivatives show moderate-to-strong antibacterial activity, with potency inversely correlated with acyl chain length. Longer chains (e.g., lauroyl) reduce solubility, limiting bioavailability .
生物活性
1,2-O-Isopropylidene-alpha-D-glucofuranose, commonly referred to as mono-acetone glucose, is a derivative of glucose that has garnered attention for its biological activities, particularly in the fields of medicinal chemistry and biochemistry. This article explores its biological activity, synthesis, and potential therapeutic applications, supported by case studies and research findings.
Chemical Structure and Synthesis
This compound is characterized by the presence of an isopropylidene group at the anomeric position of the glucose molecule. This modification enhances its stability and solubility compared to unmodified glucose. The compound can be synthesized through various methods, including selective acylation and reaction with diazomethane-stannous chloride .
Synthesis Overview
- Starting Material : D-glucose
- Reagents : Isopropylidene derivatives, acylating agents
- Methods : Direct acylation, reactions with diazomethane
Biological Activities
The biological activities of this compound include anti-inflammatory properties, immunomodulatory effects, and potential use as an antibacterial agent.
Anti-inflammatory Activity
Research indicates that derivatives of this compound exhibit significant anti-inflammatory activity. For instance, compounds derived from this sugar have shown efficacy in managing symptoms associated with rheumatoid arthritis while demonstrating low toxicity levels .
- Case Study : A study found that specific derivatives could achieve a 50% inhibition rate in cultured cells at concentrations ranging from 10 to 100 mg/ml .
Immunomodulatory Effects
These compounds have been noted for their immunomodulatory effects, potentially beneficial for treating autoimmune disorders such as psoriasis and lupus. The mechanism involves modulation of immune responses through interaction with immune cells .
Antibacterial Properties
Recent studies have evaluated the antibacterial potential of this compound and its derivatives. In vitro tests demonstrated varying degrees of antibacterial activity against common pathogens.
The mechanism through which this compound exerts its biological effects is primarily linked to its ability to interact with various enzymes and receptors involved in inflammatory pathways.
Tyrosinase Inhibition
One notable activity is the inhibition of mushroom tyrosinase, an enzyme critical in melanin biosynthesis. Inhibitory assays revealed that certain derivatives exhibit potent inhibition characteristics:
Q & A
Basic Research Questions
Q. What are the common synthetic routes for preparing 1,2-O-Isopropylidene-alpha-D-glucofuranose, and how can reaction conditions be optimized for higher yields?
The compound is typically synthesized from D-glucose via a three-step process:
- Step 1 : Acetonation of D-glucose using dry acetone, catalytic H₂SO₄, and CuSO₄ at room temperature for 24 hours, yielding bisacetone D-glucose (46% yield).
- Step 2 : Alkylation (e.g., butylation) with NaH and alkyl halides (e.g., BuBr) in THF, achieving >90% yield.
- Step 3 : Selective hydrolysis of 5,6-O-isopropylidene groups using 10% H₂SO₄ in MeOH-H₂O, followed by neutralization with K₂CO₃ (~95% yield) . Optimization includes controlling moisture (oven-dry CuSO₄), reaction time, and purification via recrystallization or chromatography. Alternative routes involve DMSO-mediated oxidation followed by NaBH₄ reduction to access stereochemical analogs .
Q. What spectroscopic techniques are most effective for characterizing this compound derivatives?
Key techniques include:
- ¹H/¹³C NMR : Assigns regioselective modifications (e.g., acylation at C-3 vs. C-6) by analyzing chemical shifts and coupling constants.
- HMBC (Heteronuclear Multiple Bond Correlation) : Resolves ambiguities in ester/ether linkage positions by correlating protons with distant carbons .
- FTIR : Identifies hydrogen-bonding patterns in self-assembled systems (e.g., organogels) and confirms functional groups (e.g., C=O stretches for esters) .
- X-ray crystallography : Provides definitive stereochemical assignments, as seen in crystal structures of phenylacetyl derivatives .
Q. How can oxidation and reduction reactions transform this compound into related carbohydrate analogs?
Oxidation with DMSO converts the C-5 hydroxyl to a ketone, forming 1,2:5,6-di-O-isopropylidene-α-D-ribo-hexofuranos-3-ulose, which is reduced with NaBH₄ to yield 1,2:5,6-di-O-isopropylidene-α-D-allofuranose. This method is scalable (>90% yield) and useful for synthesizing rare sugars .
Advanced Research Questions
Q. How can regioselective acylation or alkylation be achieved at specific hydroxyl groups of this compound?
Regioselectivity is controlled by:
- Enzymatic catalysis : Lipases (e.g., Candida antarctica) selectively acylate the C-6 hydroxyl in organic solvents, leaving C-3 and C-5 positions intact .
- Protecting group strategies : Sequential acetonation and hydrolysis isolate reactive sites. For example, 5,6-diol intermediates allow selective etherification with octyl bromide using NaH in THF (87% yield) .
- Steric and electronic effects : Bulky reagents favor less hindered positions (e.g., C-6 over C-3) .
Q. How do solvent polarity and hydrogen bonding influence the self-assembly and thermal stability of glucofuranose-based organogels?
Solvent parameters (polarity, ET(30)) dictate gelator aggregation. For example:
- Nitrobenzene (ε = 34.8) : Forms thin, rod-like fibers with ΔH = 50.1 kJ/mol.
- Chlorobenzene (ε = 5.6) : Produces thicker, curved fibers with ΔH = 65.0 kJ/mol. FTIR reveals stronger O–H···N hydrogen bonding in polar solvents, stabilizing the gel network .
Q. What strategies resolve contradictions in spectral data interpretation for structurally similar derivatives?
Conflicting NMR assignments are resolved by:
- Comparative analysis : Cross-referencing with crystallographic data (e.g., distinguishing C-3 vs. C-6 esters via X-ray structures) .
- Isotopic labeling : Using ¹³C-enriched reagents to trace regiochemical outcomes.
- Density Functional Theory (DFT) : Predicting chemical shifts for proposed structures .
Q. How do ether vs. ester linkages at C-5/C-6 impact the biological activity of glucofuranose derivatives?
Ethers (e.g., 5,6-di-O-octyl ether) exhibit higher lipophilicity, enhancing membrane permeability in antimicrobial assays. Esters (e.g., 6-O-lauroyl derivatives) show stronger inhibition of sterol 14α-demethylase due to hydrogen bonding with the enzyme’s active site .
Q. What role does molecular docking play in designing glucofuranose derivatives as antimicrobial agents or enzyme inhibitors?
Docking simulations predict binding affinities to targets like CYP51 (sterol demethylase). For example:
特性
IUPAC Name |
(1R)-1-[(3aR,5R,6S,6aR)-6-hydroxy-2,2-dimethyl-3a,5,6,6a-tetrahydrofuro[2,3-d][1,3]dioxol-5-yl]ethane-1,2-diol | |
---|---|---|
Source | PubChem | |
URL | https://pubchem.ncbi.nlm.nih.gov | |
Description | Data deposited in or computed by PubChem | |
InChI |
InChI=1S/C9H16O6/c1-9(2)14-7-5(12)6(4(11)3-10)13-8(7)15-9/h4-8,10-12H,3H2,1-2H3/t4-,5+,6-,7-,8-/m1/s1 | |
Source | PubChem | |
URL | https://pubchem.ncbi.nlm.nih.gov | |
Description | Data deposited in or computed by PubChem | |
InChI Key |
BGGCXQKYCBBHAH-OZRXBMAMSA-N | |
Source | PubChem | |
URL | https://pubchem.ncbi.nlm.nih.gov | |
Description | Data deposited in or computed by PubChem | |
Canonical SMILES |
CC1(OC2C(C(OC2O1)C(CO)O)O)C | |
Source | PubChem | |
URL | https://pubchem.ncbi.nlm.nih.gov | |
Description | Data deposited in or computed by PubChem | |
Isomeric SMILES |
CC1(O[C@@H]2[C@H]([C@H](O[C@@H]2O1)[C@@H](CO)O)O)C | |
Source | PubChem | |
URL | https://pubchem.ncbi.nlm.nih.gov | |
Description | Data deposited in or computed by PubChem | |
Molecular Formula |
C9H16O6 | |
Source | PubChem | |
URL | https://pubchem.ncbi.nlm.nih.gov | |
Description | Data deposited in or computed by PubChem | |
DSSTOX Substance ID |
DTXSID80885054 | |
Record name | .alpha.-D-Glucofuranose, 1,2-O-(1-methylethylidene)- | |
Source | EPA DSSTox | |
URL | https://comptox.epa.gov/dashboard/DTXSID80885054 | |
Description | DSSTox provides a high quality public chemistry resource for supporting improved predictive toxicology. | |
Molecular Weight |
220.22 g/mol | |
Source | PubChem | |
URL | https://pubchem.ncbi.nlm.nih.gov | |
Description | Data deposited in or computed by PubChem | |
CAS No. |
18549-40-1 | |
Record name | 1,2-O-Isopropylidene-α-D-glucofuranose | |
Source | CAS Common Chemistry | |
URL | https://commonchemistry.cas.org/detail?cas_rn=18549-40-1 | |
Description | CAS Common Chemistry is an open community resource for accessing chemical information. Nearly 500,000 chemical substances from CAS REGISTRY cover areas of community interest, including common and frequently regulated chemicals, and those relevant to high school and undergraduate chemistry classes. This chemical information, curated by our expert scientists, is provided in alignment with our mission as a division of the American Chemical Society. | |
Explanation | The data from CAS Common Chemistry is provided under a CC-BY-NC 4.0 license, unless otherwise stated. | |
Record name | 1,2-O-Isopropylidene-D-glucofuranose | |
Source | ChemIDplus | |
URL | https://pubchem.ncbi.nlm.nih.gov/substance/?source=chemidplus&sourceid=0018549401 | |
Description | ChemIDplus is a free, web search system that provides access to the structure and nomenclature authority files used for the identification of chemical substances cited in National Library of Medicine (NLM) databases, including the TOXNET system. | |
Record name | .alpha.-D-Glucofuranose, 1,2-O-(1-methylethylidene)- | |
Source | EPA Chemicals under the TSCA | |
URL | https://www.epa.gov/chemicals-under-tsca | |
Description | EPA Chemicals under the Toxic Substances Control Act (TSCA) collection contains information on chemicals and their regulations under TSCA, including non-confidential content from the TSCA Chemical Substance Inventory and Chemical Data Reporting. | |
Record name | .alpha.-D-Glucofuranose, 1,2-O-(1-methylethylidene)- | |
Source | EPA DSSTox | |
URL | https://comptox.epa.gov/dashboard/DTXSID80885054 | |
Description | DSSTox provides a high quality public chemistry resource for supporting improved predictive toxicology. | |
Record name | 1,2-O-isopropylidene-α-D-glucofuranose | |
Source | European Chemicals Agency (ECHA) | |
URL | https://echa.europa.eu/substance-information/-/substanceinfo/100.038.549 | |
Description | The European Chemicals Agency (ECHA) is an agency of the European Union which is the driving force among regulatory authorities in implementing the EU's groundbreaking chemicals legislation for the benefit of human health and the environment as well as for innovation and competitiveness. | |
Explanation | Use of the information, documents and data from the ECHA website is subject to the terms and conditions of this Legal Notice, and subject to other binding limitations provided for under applicable law, the information, documents and data made available on the ECHA website may be reproduced, distributed and/or used, totally or in part, for non-commercial purposes provided that ECHA is acknowledged as the source: "Source: European Chemicals Agency, http://echa.europa.eu/". Such acknowledgement must be included in each copy of the material. ECHA permits and encourages organisations and individuals to create links to the ECHA website under the following cumulative conditions: Links can only be made to webpages that provide a link to the Legal Notice page. | |
Retrosynthesis Analysis
AI-Powered Synthesis Planning: Our tool employs the Template_relevance Pistachio, Template_relevance Bkms_metabolic, Template_relevance Pistachio_ringbreaker, Template_relevance Reaxys, Template_relevance Reaxys_biocatalysis model, leveraging a vast database of chemical reactions to predict feasible synthetic routes.
One-Step Synthesis Focus: Specifically designed for one-step synthesis, it provides concise and direct routes for your target compounds, streamlining the synthesis process.
Accurate Predictions: Utilizing the extensive PISTACHIO, BKMS_METABOLIC, PISTACHIO_RINGBREAKER, REAXYS, REAXYS_BIOCATALYSIS database, our tool offers high-accuracy predictions, reflecting the latest in chemical research and data.
Strategy Settings
Precursor scoring | Relevance Heuristic |
---|---|
Min. plausibility | 0.01 |
Model | Template_relevance |
Template Set | Pistachio/Bkms_metabolic/Pistachio_ringbreaker/Reaxys/Reaxys_biocatalysis |
Top-N result to add to graph | 6 |
Feasible Synthetic Routes
試験管内研究製品の免責事項と情報
BenchChemで提示されるすべての記事および製品情報は、情報提供を目的としています。BenchChemで購入可能な製品は、生体外研究のために特別に設計されています。生体外研究は、ラテン語の "in glass" に由来し、生物体の外で行われる実験を指します。これらの製品は医薬品または薬として分類されておらず、FDAから任何の医療状態、病気、または疾患の予防、治療、または治癒のために承認されていません。これらの製品を人間または動物に体内に導入する形態は、法律により厳格に禁止されています。これらのガイドラインに従うことは、研究と実験において法的および倫理的な基準の遵守を確実にするために重要です。