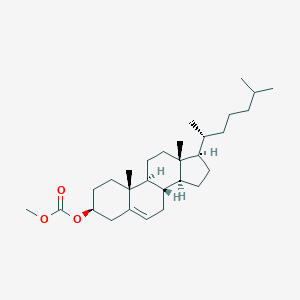
Cholesteryl methyl carbonate
概要
説明
Cholesteryl methyl carbonate is a chemical compound with the molecular formula C29H48O3 and a molecular weight of 444.6896 . . This compound is derived from cholesterol and is characterized by the presence of a carbonate ester functional group.
科学的研究の応用
Cholesteryl methyl carbonate has a wide range of applications in scientific research, including:
作用機序
Target of Action
Cholesteryl methyl carbonate (CMC) is a derivative of cholesterol, a vital molecule in the body. The primary targets of CMC are cholesteryl ester transfer proteins (CETP) . CETP plays a key role in reverse cholesterol transport (RCT) by mediating the transfer of cholesteryl esters (CEs) and triglycerides (TGs) between high-density lipoproteins (HDLs) and apolipoprotein B-100 (apoB-100) containing lipoproteins in plasma .
Mode of Action
The interaction of CMC with its targets involves the transfer of cholesteryl esters (CEs) among lipoproteins . This process is regulated by CETP, which can be inhibited by certain molecules, potentially including CMC .
Biochemical Pathways
The action of C correctly. The action of CMC affects the cholesterol homeostasis in the body. This homeostasis reflects the dynamic balance between cholesterol biosynthesis, uptake, export, and esterification . Esterification is a process in which cholesterol is converted to neutral cholesteryl esters . Disturbances in this balance can lead to various diseases, such as cardiovascular diseases, neurodegenerative diseases, and cancers .
Pharmacokinetics
It is known that the compound’s long-circulating and ph-sensitive properties can be enhanced by constructing bifunctional liposomes using poly(2-ethyl-oxazoline)-cholesteryl methyl carbonate (petoz-chmc) .
Result of Action
The action of CMC can lead to changes in the levels of low-density lipoprotein cholesterol (LDL-C) and high-density lipoprotein cholesterol (HDL-C) in the plasma . These changes can affect the risk of atherosclerotic cardiovascular disease (ASCVD) .
Action Environment
The action, efficacy, and stability of CMC can be influenced by various environmental factors. For instance, the pH level can affect the fusion and cellular uptake of liposomes modified with PEtOz-CHMC . More research is needed to fully understand how other environmental factors may influence the action of CMC.
生化学分析
Biochemical Properties
The biochemical properties of CMC are not well-studied. As a derivative of cholesterol, it may interact with various biomolecules. Cholesterol is known to interact with a variety of proteins, including those involved in lipid metabolism and transport . It’s plausible that CMC may have similar interactions, but specific enzymes, proteins, or other biomolecules that interact with CMC are not currently known.
Cellular Effects
Cholesterol, from which CMC is derived, plays a crucial role in maintaining cell membrane integrity and fluidity . It’s possible that CMC may have similar effects on cell function, but this has not been confirmed.
Metabolic Pathways
Cholesterol, the parent compound of CMC, is involved in several metabolic pathways, including the synthesis of steroid hormones . It’s possible that CMC may be involved in similar pathways, but this has not been confirmed.
Transport and Distribution
Cholesterol, from which CMC is derived, is transported and distributed within cells and tissues by various transporters and binding proteins . It’s plausible that CMC may have similar transport and distribution mechanisms, but this has not been confirmed.
Subcellular Localization
Cholesterol, the parent compound of CMC, is predominantly localized in the plasma membrane of cells . It’s possible that CMC may have a similar subcellular localization, but this has not been confirmed.
準備方法
Synthetic Routes and Reaction Conditions: Cholesteryl methyl carbonate can be synthesized through the reaction of cholesterol with methyl chloroformate in the presence of a base such as pyridine . The reaction typically proceeds under mild conditions, with the base acting as a catalyst to facilitate the formation of the carbonate ester linkage.
Industrial Production Methods: In an industrial setting, the production of this compound may involve the use of large-scale reactors and continuous flow processes to ensure high yield and purity. The reaction conditions are optimized to minimize side reactions and maximize the efficiency of the carbonate ester formation.
化学反応の分析
Types of Reactions: Cholesteryl methyl carbonate undergoes various chemical reactions, including:
Hydrolysis: The carbonate ester group can be hydrolyzed to yield cholesterol and methanol under acidic or basic conditions.
Transesterification: This reaction involves the exchange of the methyl group with another alcohol, resulting in the formation of different cholesteryl esters.
Oxidation: The compound can undergo oxidation reactions, leading to the formation of oxidized derivatives of cholesterol.
Common Reagents and Conditions:
Hydrolysis: Acidic or basic catalysts such as hydrochloric acid or sodium hydroxide.
Transesterification: Alcohols and catalysts such as sodium methoxide.
Oxidation: Oxidizing agents like potassium permanganate or chromium trioxide.
Major Products Formed:
Hydrolysis: Cholesterol and methanol.
Transesterification: Various cholesteryl esters.
Oxidation: Oxidized cholesterol derivatives.
類似化合物との比較
Cholesteryl oleyl carbonate: Another cholesterol derivative with a carbonate ester group, used in liquid crystal applications.
Cholesteryl butyrate: A cholesteryl ester with a shorter chain fatty acid, used in studies of lipid metabolism.
Cholesteryl acetate: A simple cholesteryl ester, often used as a reference compound in analytical studies.
Uniqueness of Cholesteryl Methyl Carbonate: this compound is unique due to its specific carbonate ester functional group, which imparts distinct chemical reactivity and physical properties. This makes it particularly useful in the synthesis of other cholesterol derivatives and in applications requiring precise control over esterification reactions.
特性
IUPAC Name |
[10,13-dimethyl-17-(6-methylheptan-2-yl)-2,3,4,7,8,9,11,12,14,15,16,17-dodecahydro-1H-cyclopenta[a]phenanthren-3-yl] methyl carbonate | |
---|---|---|
Details | Computed by Lexichem TK 2.7.0 (PubChem release 2021.05.07) | |
Source | PubChem | |
URL | https://pubchem.ncbi.nlm.nih.gov | |
Description | Data deposited in or computed by PubChem | |
InChI |
InChI=1S/C29H48O3/c1-19(2)8-7-9-20(3)24-12-13-25-23-11-10-21-18-22(32-27(30)31-6)14-16-28(21,4)26(23)15-17-29(24,25)5/h10,19-20,22-26H,7-9,11-18H2,1-6H3 | |
Details | Computed by InChI 1.0.6 (PubChem release 2021.05.07) | |
Source | PubChem | |
URL | https://pubchem.ncbi.nlm.nih.gov | |
Description | Data deposited in or computed by PubChem | |
InChI Key |
WHMGDIMLSAAHJQ-UHFFFAOYSA-N | |
Details | Computed by InChI 1.0.6 (PubChem release 2021.05.07) | |
Source | PubChem | |
URL | https://pubchem.ncbi.nlm.nih.gov | |
Description | Data deposited in or computed by PubChem | |
Canonical SMILES |
CC(C)CCCC(C)C1CCC2C1(CCC3C2CC=C4C3(CCC(C4)OC(=O)OC)C)C | |
Details | Computed by OEChem 2.3.0 (PubChem release 2021.05.07) | |
Source | PubChem | |
URL | https://pubchem.ncbi.nlm.nih.gov | |
Description | Data deposited in or computed by PubChem | |
Molecular Formula |
C29H48O3 | |
Details | Computed by PubChem 2.1 (PubChem release 2021.05.07) | |
Source | PubChem | |
URL | https://pubchem.ncbi.nlm.nih.gov | |
Description | Data deposited in or computed by PubChem | |
DSSTOX Substance ID |
DTXSID00935126 | |
Record name | Cholest-5-en-3-yl methyl carbonate | |
Source | EPA DSSTox | |
URL | https://comptox.epa.gov/dashboard/DTXSID00935126 | |
Description | DSSTox provides a high quality public chemistry resource for supporting improved predictive toxicology. | |
Molecular Weight |
444.7 g/mol | |
Details | Computed by PubChem 2.1 (PubChem release 2021.05.07) | |
Source | PubChem | |
URL | https://pubchem.ncbi.nlm.nih.gov | |
Description | Data deposited in or computed by PubChem | |
CAS No. |
15507-52-5 | |
Record name | Cholest-5-en-3-ol (3beta)-, 3-(methyl carbonate) | |
Source | ChemIDplus | |
URL | https://pubchem.ncbi.nlm.nih.gov/substance/?source=chemidplus&sourceid=0015507525 | |
Description | ChemIDplus is a free, web search system that provides access to the structure and nomenclature authority files used for the identification of chemical substances cited in National Library of Medicine (NLM) databases, including the TOXNET system. | |
Record name | Cholest-5-en-3-yl methyl carbonate | |
Source | EPA DSSTox | |
URL | https://comptox.epa.gov/dashboard/DTXSID00935126 | |
Description | DSSTox provides a high quality public chemistry resource for supporting improved predictive toxicology. | |
Retrosynthesis Analysis
AI-Powered Synthesis Planning: Our tool employs the Template_relevance Pistachio, Template_relevance Bkms_metabolic, Template_relevance Pistachio_ringbreaker, Template_relevance Reaxys, Template_relevance Reaxys_biocatalysis model, leveraging a vast database of chemical reactions to predict feasible synthetic routes.
One-Step Synthesis Focus: Specifically designed for one-step synthesis, it provides concise and direct routes for your target compounds, streamlining the synthesis process.
Accurate Predictions: Utilizing the extensive PISTACHIO, BKMS_METABOLIC, PISTACHIO_RINGBREAKER, REAXYS, REAXYS_BIOCATALYSIS database, our tool offers high-accuracy predictions, reflecting the latest in chemical research and data.
Strategy Settings
Precursor scoring | Relevance Heuristic |
---|---|
Min. plausibility | 0.01 |
Model | Template_relevance |
Template Set | Pistachio/Bkms_metabolic/Pistachio_ringbreaker/Reaxys/Reaxys_biocatalysis |
Top-N result to add to graph | 6 |
Feasible Synthetic Routes
Q1: What are the structural characteristics of Cholesteryl methyl carbonate?
A1: this compound (C29H48O3) is a cholesterogen with a molecular weight of 444.69 g/mol. [] Its crystal structure has been determined using X-ray diffraction, revealing it crystallizes in the monoclinic space group P21. []
Q2: What mesomorphic properties does this compound exhibit?
A2: this compound displays cholesteric mesophases and exhibits cholesteric colors of the platelet type. [] This means it forms liquid crystal phases characterized by a helical arrangement of molecules.
Q3: How does the alkyl chain length in cholesteryl alkyl carbonates influence their mesomorphic behavior?
A3: Increasing alkyl chain length in homologous cholesteryl alkyl carbonates generally leads to higher transition temperatures for both cholesteric and smectic mesophases. [] This trend is typical for sterols exhibiting these mesophases.
Q4: Can you elaborate on the "blue phase" observed in this compound and similar cholesteric liquid crystals?
A4: The "blue phase," observed in this compound, cholesteryl benzoate, cholesteryl ethyl carbonate, and cholesteryl laurate, exhibits NMR spectra resembling the isotropic phase, albeit with a broader line indicating long-range orientational order. [] This suggests a structure distinct from both the ordinary cholesteric and isotropic phases.
Q5: How has infrared spectroscopy contributed to understanding the structure of this compound and its analogs?
A5: Infrared spectroscopy studies on cholesteryl alkyl carbonates, including this compound, have provided insights into the molecular structure and interactions. [] For instance, shifts in the carbonyl frequency in cholesteryl oleyl carbonate have been linked to changes in coplanarity with the benzene ring.
Q6: What are the potential applications of this compound in drug delivery?
A6: this compound is utilized in the synthesis of biocompatible polymers like poly(2-ethyl-2-oxazoline)-cholesteryl methyl carbonate (PEOz-CHMC). [, , , ] This polymer can modify liposomes, enhancing their circulation time, pH-sensitivity, and ability to escape endosomes, making them promising for targeted drug delivery. [, , , ]
Q7: How does the molecular weight of PEOz-CHMC affect the properties of modified liposomes?
A7: Research indicates that both the molecular weight and molar ratio of PEOz-CHMC significantly influence the pH sensitivity, stability, and antitumor efficacy of liposomes. [] Specifically, PEOz-CHMC with molecular weights of 1 kDa and 2 kDa at specific molar ratios exhibited optimal pH responsiveness and enhanced stability. []
Q8: Can you provide an example of how PEOz-CHMC modified liposomes were tested for antitumor efficacy?
A8: PEOz-CHMC modified liposomes loaded with doxorubicin hydrochloride (DOX x HCl) demonstrated enhanced antitumor activity against B16 tumor xenograft models in vivo compared to unmodified liposomes. [] This highlights the potential of this modification strategy for improving cancer treatment.
Q9: What role does the buffering capacity of PEOz-CHMC play in drug delivery?
A9: The buffering capacity of PEOz-CHMC contributes to the pH-sensitive release of encapsulated drugs from liposomes. [] This is particularly important for drugs with optimal activity in acidic environments, like within tumor cells.
Q10: What is the significance of studying gamma-irradiated this compound?
A10: Gamma irradiation of this compound allows researchers to investigate the formation and behavior of free radicals within the material. [, ] Electron spin resonance (EPR) studies on irradiated crystals have provided insights into the nature and properties of these radicals. []
試験管内研究製品の免責事項と情報
BenchChemで提示されるすべての記事および製品情報は、情報提供を目的としています。BenchChemで購入可能な製品は、生体外研究のために特別に設計されています。生体外研究は、ラテン語の "in glass" に由来し、生物体の外で行われる実験を指します。これらの製品は医薬品または薬として分類されておらず、FDAから任何の医療状態、病気、または疾患の予防、治療、または治癒のために承認されていません。これらの製品を人間または動物に体内に導入する形態は、法律により厳格に禁止されています。これらのガイドラインに従うことは、研究と実験において法的および倫理的な基準の遵守を確実にするために重要です。