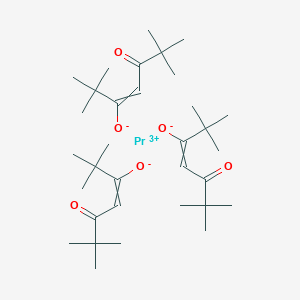
トリス(2,2,6,6-テトラメチルヘプタン-3,5-ジオナト-O,O')プラセオジム
- 専門家チームからの見積もりを受け取るには、QUICK INQUIRYをクリックしてください。
- 品質商品を競争力のある価格で提供し、研究に集中できます。
概要
説明
科学的研究の応用
Tris(dipivalomethanato)praseodymium has several scientific research applications:
作用機序
Target of Action
Tris(2,2,6,6-tetramethylheptane-3,5-dionato-O,O’)praseodymium, also known as Praseodymium(III)-DPM, is a complex compound that primarily targets lanthanide ions . The compound is used as a ligand, which binds to these ions and forms stable complexes .
Mode of Action
The compound interacts with its targets through a process known as chelation. It acts as a bidentate ligand, meaning it can bind to a metal ion at two distinct points . This interaction results in the formation of a ring-like structure, which is characteristic of chelation. The compound is also known to undergo O-additions and C-additions .
Biochemical Pathways
It is known that the compound can act as an air-stable ligand for metal catalysts in various reactions . This suggests that it may play a role in catalyzing certain biochemical reactions.
Pharmacokinetics
It is known that the compound is a stable, anhydrous reagent , which suggests that it may have good stability and could potentially be absorbed and distributed in the body
Result of Action
It is known that the compound can improve the luminescence properties of gan light-emitting diodes when used as a doping agent . This suggests that it may have potential applications in optoelectronics.
Action Environment
The action of Tris(2,2,6,6-tetramethylheptane-3,5-dionato-O,O’)praseodymium can be influenced by environmental factors. For instance, it is known to be sensitive to light, air, and moisture . Therefore, it is typically stored under inert gas to maintain its stability . The compound’s efficacy may also be affected by temperature, as suggested by a study on a similar compound, Tris(2,2,6,6-tetramethylheptan-3,5-dionato)yttrium(III), which found that the compound’s molecular forms in the gas phase can change with temperature .
生化学分析
Biochemical Properties
Similar compounds have been found to act as ligands for metal catalysts . These compounds can undergo O-additions and C-additions, indicating that they may interact with various enzymes, proteins, and other biomolecules .
Molecular Mechanism
It has been suggested that similar compounds may exert their effects at the molecular level through binding interactions with biomolecules, enzyme inhibition or activation, and changes in gene expression .
準備方法
The synthesis of tris(dipivalomethanato)praseodymium typically involves the reaction of praseodymium chloride with dipivaloylmethane in the presence of a base such as sodium hydroxide. The reaction is carried out in an organic solvent like ethanol or acetone under reflux conditions. The product is then purified by recrystallization .
Industrial production methods for this compound are similar but often involve larger scale reactions and more rigorous purification processes to ensure high purity and yield .
化学反応の分析
Tris(dipivalomethanato)praseodymium undergoes various chemical reactions, including:
Oxidation: This compound can be oxidized under specific conditions, although detailed studies on its oxidation reactions are limited.
Reduction: It can be reduced by strong reducing agents, leading to the formation of praseodymium metal or lower oxidation state complexes.
Common reagents used in these reactions include strong acids, bases, and reducing agents. The major products formed depend on the specific reaction conditions and reagents used.
類似化合物との比較
Tris(dipivalomethanato)praseodymium is similar to other lanthanide tris(dipivalomethanato) complexes, such as those of europium and samarium. it is unique due to the specific paramagnetic properties of praseodymium, which result in distinct NMR shift patterns .
Similar Compounds
- Tris(dipivalomethanato)europium
- Tris(dipivalomethanato)samarium
- Tris(dipivalomethanato)neodymium
These compounds share similar structures but differ in their magnetic properties and the extent of the shifts they induce in NMR spectra.
生物活性
Tris(dipivalomethanato)praseodymium (Pr(DPM)₃) is a lanthanide complex that has garnered attention in various fields of chemical and biological research due to its unique properties and potential applications. This article provides a comprehensive overview of the biological activity associated with Pr(DPM)₃, drawing on diverse sources to present a detailed analysis.
Chemical Structure and Properties
Tris(dipivalomethanato)praseodymium is characterized by its coordination structure, where praseodymium is coordinated to three dipivalomethanate ligands. The molecular formula for this compound is C₃₃H₅₇O₆Pr, and it exhibits significant stability and solubility in organic solvents, which enhances its utility in biological studies.
Chirality Determination
One of the primary biological applications of Pr(DPM)₃ is in the determination of chirality in organic compounds. Research indicates that this complex can effectively differentiate between enantiomers of amines and cyclic amino alcohols through induced shifts in NMR spectroscopy. For instance, a study published in the Journal of the American Chemical Society demonstrated that Pr(DPM)₃ can be used as a chiral shift reagent to determine the absolute configuration of vicinal glycols and amino alcohols .
Table 1: Summary of Biological Activities Associated with Pr(DPM)₃
The mechanism by which Pr(DPM)₃ exerts its biological effects primarily involves its ability to interact with specific molecular structures, leading to observable changes in NMR spectra. The lanthanide ion's unique electronic properties allow it to induce significant chemical shifts that are sensitive to the spatial arrangement of atoms within chiral molecules.
Case Studies
Several studies have highlighted the effectiveness of Pr(DPM)₃ in various biological contexts:
- Chiral Amines and Alcohols : A systematic study focused on the use of Pr(DPM)₃ as a chiral shift reagent revealed its capacity to distinguish between different stereoisomers effectively. The study provided quantitative data on the degree of shift observed for various substrates, establishing a reliable method for chirality determination .
- Carbohydrate Analysis : Another investigation explored how Pr(DPM)₃ influences the NMR spectra of carbohydrates, demonstrating its utility in elucidating structural features and conformational dynamics within these biologically relevant molecules .
- Enantiomeric Ratios : Research has also shown that Pr(DPM)₃ can be employed to analyze enantiomeric compositions of chiral compounds, providing insights into their potential biological activities and interactions .
特性
CAS番号 |
15492-48-5 |
---|---|
分子式 |
C33H60O6Pr |
分子量 |
693.7 g/mol |
IUPAC名 |
(Z)-5-hydroxy-2,2,6,6-tetramethylhept-4-en-3-one;praseodymium |
InChI |
InChI=1S/3C11H20O2.Pr/c3*1-10(2,3)8(12)7-9(13)11(4,5)6;/h3*7,12H,1-6H3;/b3*8-7-; |
InChIキー |
KSKFOHQCACWEIV-LWTKGLMZSA-N |
SMILES |
CC(C)(C)C(=CC(=O)C(C)(C)C)[O-].CC(C)(C)C(=CC(=O)C(C)(C)C)[O-].CC(C)(C)C(=CC(=O)C(C)(C)C)[O-].[Pr+3] |
異性体SMILES |
CC(/C(=C/C(=O)C(C)(C)C)/O)(C)C.CC(/C(=C/C(=O)C(C)(C)C)/O)(C)C.CC(/C(=C/C(=O)C(C)(C)C)/O)(C)C.[Pr] |
正規SMILES |
CC(C)(C)C(=CC(=O)C(C)(C)C)O.CC(C)(C)C(=CC(=O)C(C)(C)C)O.CC(C)(C)C(=CC(=O)C(C)(C)C)O.[Pr] |
Key on ui other cas no. |
15492-48-5 |
ピクトグラム |
Irritant |
製品の起源 |
United States |
Retrosynthesis Analysis
AI-Powered Synthesis Planning: Our tool employs the Template_relevance Pistachio, Template_relevance Bkms_metabolic, Template_relevance Pistachio_ringbreaker, Template_relevance Reaxys, Template_relevance Reaxys_biocatalysis model, leveraging a vast database of chemical reactions to predict feasible synthetic routes.
One-Step Synthesis Focus: Specifically designed for one-step synthesis, it provides concise and direct routes for your target compounds, streamlining the synthesis process.
Accurate Predictions: Utilizing the extensive PISTACHIO, BKMS_METABOLIC, PISTACHIO_RINGBREAKER, REAXYS, REAXYS_BIOCATALYSIS database, our tool offers high-accuracy predictions, reflecting the latest in chemical research and data.
Strategy Settings
Precursor scoring | Relevance Heuristic |
---|---|
Min. plausibility | 0.01 |
Model | Template_relevance |
Template Set | Pistachio/Bkms_metabolic/Pistachio_ringbreaker/Reaxys/Reaxys_biocatalysis |
Top-N result to add to graph | 6 |
Feasible Synthetic Routes
試験管内研究製品の免責事項と情報
BenchChemで提示されるすべての記事および製品情報は、情報提供を目的としています。BenchChemで購入可能な製品は、生体外研究のために特別に設計されています。生体外研究は、ラテン語の "in glass" に由来し、生物体の外で行われる実験を指します。これらの製品は医薬品または薬として分類されておらず、FDAから任何の医療状態、病気、または疾患の予防、治療、または治癒のために承認されていません。これらの製品を人間または動物に体内に導入する形態は、法律により厳格に禁止されています。これらのガイドラインに従うことは、研究と実験において法的および倫理的な基準の遵守を確実にするために重要です。