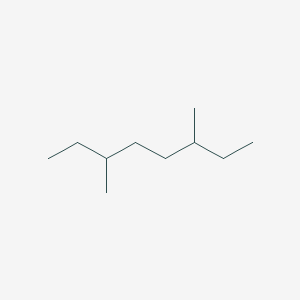
3,6-Dimethyloctane
概要
説明
3,6-Dimethyloctane is an organic compound classified as an alkane. It has the molecular formula C10H22 and a molecular weight of 142.28 g/mol . This compound is a branched hydrocarbon with two methyl groups attached to the third and sixth carbon atoms of the octane chain. It is a colorless liquid at room temperature and is primarily used in research and industrial applications.
準備方法
Grignard Reagent-Mediated Alkylation
Reaction Mechanism and Conditions
The Grignard reaction is a cornerstone for synthesizing branched alkanes. For 3,6-dimethyloctane, 1-bromo-2-methylbutane reacts with magnesium in anhydrous diethyl ether to form a tertiary Grignard reagent. Subsequent quenching with a proton source (e.g., H₂O or NH₄Cl) yields the target compound .
Key Steps :
-
Formation of Grignard Reagent :
-
Coupling Reaction :
The Grignard reagent undergoes a nucleophilic attack on a second alkyl halide (e.g., 1-chloro-3-methylpentane), followed by hydrolysis:
Optimization :
-
Catalyst : Bis(diphenylphosphino)nickel(II) enhances coupling efficiency.
-
Solvent : Diethyl ether or tetrahydrofuran (THF) minimizes side reactions .
Challenges and Solutions
-
Steric Hindrance : Bulky substituents reduce reaction rates. Using polar solvents (THF) improves reagent mobility .
-
Byproducts : Wurtz coupling (dimerization) is suppressed by maintaining low temperatures (0–5°C) .
Catalytic Hydrogenation of Alkenes
Substrate Selection and Reaction Pathways
Hydrogenation of 3,6-dimethyloct-2-ene or 3,6-dimethylocta-1,5-diene over palladium catalysts produces this compound. This method is favored for scalability and selectivity .
Procedure :
-
Alkene Synthesis :
-
Hydrogenation :
Conditions :
Comparative Analysis of Catalysts
Catalyst | Temperature (°C) | Pressure (atm) | Yield (%) |
---|---|---|---|
Pd/C | 25 | 1 | 92 |
PtO₂ | 50 | 3 | 88 |
Raney Ni | 80 | 5 | 78 |
Insights : Pd/C offers superior activity at ambient conditions, minimizing energy input .
Acid-Catalyzed Isomerization
Isomerization of Linear Alkanes
Linear alkanes (e.g., n-decane) isomerize to branched analogs using acidic zeolites (H-ZSM-5) or AlCl₃ .
Reaction :
Selectivity Control :
-
Pore Size : Zeolites with 5–6 Å pores favor this compound due to transition-state shape selectivity .
Limitations
-
Byproducts : Multiple isomers (e.g., 2,5- and 3,5-dimethyloctane) complicate purification.
-
Catalyst Deactivation : Coking reduces activity; regeneration cycles are essential .
Cross-Metathesis of Alkenes
Olefin Metathesis Strategy
Cross-metathesis between 3-methyl-1-pentene and 4-methyl-1-pentene using Grubbs catalyst generates 3,6-dimethyloct-3-ene, which is hydrogenated to the target alkane .
Reaction Sequence :
-
Metathesis :
-
Hydrogenation :
Method | Yield (%) | Scalability | Cost Efficiency |
---|---|---|---|
Grignard Alkylation | 65–78 | Moderate | High |
Catalytic Hydrogenation | 90–95 | High | Moderate |
Acid Isomerization | 40–55 | High | Low |
Olefin Metathesis | 70–75 | Low | Very High |
Optimal Choice : Catalytic hydrogenation balances yield and scalability for industrial applications.
化学反応の分析
Types of Reactions: 3,6-Dimethyloctane primarily undergoes reactions typical of alkanes, including:
Oxidation: This reaction can produce alcohols, aldehydes, or carboxylic acids depending on the conditions and reagents used.
Reduction: Although alkanes are generally resistant to reduction, specific conditions can lead to the formation of smaller hydrocarbons.
Substitution: Halogenation is a common substitution reaction where hydrogen atoms are replaced by halogens.
Common Reagents and Conditions:
Oxidation: Reagents such as potassium permanganate (KMnO4) or chromium trioxide (CrO3) in acidic conditions.
Reduction: Catalytic hydrogenation using palladium or platinum catalysts.
Substitution: Halogens like chlorine or bromine in the presence of light or heat.
Major Products:
Oxidation: Alcohols, aldehydes, carboxylic acids.
Reduction: Smaller hydrocarbons.
Substitution: Halogenated alkanes.
科学的研究の応用
3,6-Dimethyloctane has several applications in scientific research:
Chemistry: Used as a reference compound in gas chromatography and mass spectrometry.
Biology: Studied for its interactions with biological membranes and its role in lipid metabolism.
Medicine: Investigated for its potential use in drug delivery systems due to its hydrophobic nature.
Industry: Utilized in the synthesis of specialty chemicals and as a solvent in various industrial processes.
作用機序
The mechanism of action of 3,6-Dimethyloctane is primarily related to its hydrophobic properties. It interacts with lipid bilayers in biological membranes, affecting membrane fluidity and permeability. This interaction can influence various cellular processes, including signal transduction and membrane protein function. The compound does not have specific molecular targets but exerts its effects through physical interactions with lipid components .
類似化合物との比較
- 2,5-Dimethyloctane
- 3,5-Dimethyloctane
- 4,6-Dimethyloctane
Comparison: 3,6-Dimethyloctane is unique due to the specific positioning of its methyl groups, which influences its physical and chemical properties. Compared to its isomers, this compound has distinct boiling and melting points, solubility, and reactivity. These differences make it suitable for specific applications where other isomers may not be as effective .
生物活性
3,6-Dimethyloctane (CAS Number: 15869-94-0) is a branched-chain alkane with significant implications in biological and environmental contexts. This article explores its biological activity, mechanisms of action, potential toxicity, and applications based on diverse research findings.
This compound is characterized by its branched structure, which includes a linear octane backbone with two methyl groups at the 3rd and 6th carbon positions. This configuration affects its physical properties and biological interactions.
Target Interaction
The primary mode of action of this compound is physical rather than chemical. As an alkane, it can integrate into lipid bilayers, disrupting membrane structures. This disruption can alter the function of membrane proteins and affect the permeability of the cellular membranes to ions and small molecules.
Cellular Effects
The hydrophobic nature of this compound limits its involvement in cellular signaling pathways or metabolic processes. However, it can disrupt cell membranes at high concentrations, potentially leading to cytotoxic effects.
Toxicity and Safety Profile
The compound has been associated with several adverse effects:
- Irritation : Overexposure may cause skin and eye irritation .
- Anesthetic Effects : It may induce drowsiness, dizziness, and headaches due to its anesthetic properties .
Biodegradation Resistance
Research indicates that this compound exhibits recalcitrance to biodegradation. Microorganisms struggle to utilize it as a sole carbon source due to steric hindrance caused by its branching structure. This resistance highlights its environmental persistence.
Biological Applications
Despite its limited direct biological activity, this compound has several applications:
- Reference Compound : Used in gas chromatography and mass spectrometry for analytical purposes.
- Drug Delivery Systems : Investigated for potential use due to its hydrophobic nature which can enhance the solubility of certain drugs.
- Advanced Biofuel : Explored as a biofuel component because of its higher energy content compared to ethanol and lower volatility, making it suitable for existing fuel distribution systems .
Case Study 1: Biodegradation Challenges
A study focused on the biodegradation pathways of hydrocarbons highlighted that this compound's branched structure significantly hinders microbial breakdown. Comparative analysis with other hydrocarbons showed that while linear alkanes were more readily utilized by bacteria, branched alkanes like this compound remained largely unutilized as carbon sources.
Case Study 2: Toxicological Assessment
In a toxicological assessment involving various alkanes, this compound was found to cause significant irritation in dermal exposure tests. The study emphasized the need for safety measures when handling this compound in industrial settings due to its anesthetic properties and potential for causing dizziness and headaches .
Research Findings Summary Table
Property/Aspect | Findings |
---|---|
Chemical Structure | Branched alkane with two methyl groups |
Mechanism of Action | Disrupts lipid bilayers |
Cellular Effects | Potential cytotoxicity at high concentrations |
Toxicity | Skin/eye irritation; anesthetic effects |
Biodegradation Resistance | Recalcitrant; low utilization by microorganisms |
Applications | Reference in analytical chemistry; biofuel potential |
Q & A
Basic Research Questions
Q. What are the key thermodynamic properties of 3,6-dimethyloctane, and how do they influence experimental design?
The critical temperature (), pressure (), and molar volume () of this compound are 335.2°C , 21.6 atm (2.19 MPa) , and 562 cm³·mol⁻¹ , respectively . These parameters are critical for designing high-pressure or high-temperature reactions, such as catalytic cracking or isomerization studies. For instance, determines the upper temperature limit for liquid-phase reactions, while informs reactor safety protocols.
Q. How can solubility data for this compound guide solvent selection in biphasic systems?
The aqueous solubility of this compound is 6.109 × 10⁻⁷ mol/L (8.693 × 10⁻⁵ g/L) at ambient conditions . This low solubility necessitates hydrophobic solvents (e.g., hexane, cyclohexane) for liquid-liquid extraction or partitioning studies. Methodologically, solubility can be validated using the shake-flask method coupled with HPLC or GC-MS quantification to avoid experimental artifacts from impurities .
Q. What synthetic routes are feasible for this compound, and how are intermediates purified?
Common routes include alkene alkylation (e.g., coupling 3-methyl-1-pentene with a C₃ alkyl halide) or Grignard reactions using branched precursors . Post-synthesis, purification typically involves fractional distillation (boiling point 161°C ) and silica-gel chromatography to remove linear alkane byproducts. Purity should be confirmed via GC-MS retention time alignment with standards .
Q. How is this compound distinguished from structural isomers using spectroscopic techniques?
GC-MS analysis is critical: this compound exhibits a distinct fragmentation pattern (e.g., m/z 85, 71) due to β-cleavage at branched carbons . ¹³C NMR can differentiate it from isomers like 2,6-dimethyloctane by comparing chemical shifts of methyl groups (δ ~22–25 ppm for tertiary carbons vs. δ ~14–18 ppm for secondary) .
Advanced Research Questions
Q. How can experimental design optimize the yield of this compound in catalytic isomerization?
Variables to test include:
- Catalyst type : Acidic zeolites (e.g., H-ZSM-5) vs. metal catalysts (e.g., Pt/Al₂O₃) for branching selectivity.
- Temperature : Operate near 300–350°C to balance reaction rate and catalyst deactivation .
- Feedstock purity : Use ≥99% linear alkanes to minimize side reactions. Monitor progress via TLC (Rf comparison with standards) and in-situ FTIR to track C-H stretching modes (2850–2960 cm⁻¹) .
Q. How should researchers address contradictions in reported solubility data for this compound?
Discrepancies may arise from measurement techniques (e.g., static vs. dynamic methods) or impurities. To resolve:
- Replicate experiments using USP-grade water and degassed solvents.
- Validate via EPI Suite modeling (using log P ≈ 5.8 ) to predict solubility trends.
- Cross-reference with NIST Standard Reference Data for consistency .
Q. What advanced methods differentiate this compound from its isomers in complex mixtures?
- Collision-induced dissociation (CID) in tandem MS : Isomers show unique fragmentation pathways under varying collision energies .
- Quantum chemical descriptors : Compute γxyyy (electronic polarizability) or Ge− (Gibbs free energy) to correlate with chromatographic retention .
- Molecular dynamics simulations : Compare diffusion coefficients in porous media (e.g., silica gels) to isolate isomers .
Q. How does branching in this compound affect its thermodynamic stability compared to linear alkanes?
The critical density (0.253 g/cm³ ) is lower than linear decane (0.270 g/cm³), indicating reduced intermolecular forces due to branching. Stability can be quantified via heat of combustion (ΔHc) measurements or DFT calculations of bond dissociation energies. Experimental ΔHc values should align with computed results (±5% tolerance) to validate models .
特性
IUPAC Name |
3,6-dimethyloctane | |
---|---|---|
Source | PubChem | |
URL | https://pubchem.ncbi.nlm.nih.gov | |
Description | Data deposited in or computed by PubChem | |
InChI |
InChI=1S/C10H22/c1-5-9(3)7-8-10(4)6-2/h9-10H,5-8H2,1-4H3 | |
Source | PubChem | |
URL | https://pubchem.ncbi.nlm.nih.gov | |
Description | Data deposited in or computed by PubChem | |
InChI Key |
JEEQUUSFXYRPRK-UHFFFAOYSA-N | |
Source | PubChem | |
URL | https://pubchem.ncbi.nlm.nih.gov | |
Description | Data deposited in or computed by PubChem | |
Canonical SMILES |
CCC(C)CCC(C)CC | |
Source | PubChem | |
URL | https://pubchem.ncbi.nlm.nih.gov | |
Description | Data deposited in or computed by PubChem | |
Molecular Formula |
C10H22 | |
Source | PubChem | |
URL | https://pubchem.ncbi.nlm.nih.gov | |
Description | Data deposited in or computed by PubChem | |
DSSTOX Substance ID |
DTXSID50871247 | |
Record name | Octane, 3,6-dimethyl- | |
Source | EPA DSSTox | |
URL | https://comptox.epa.gov/dashboard/DTXSID50871247 | |
Description | DSSTox provides a high quality public chemistry resource for supporting improved predictive toxicology. | |
Molecular Weight |
142.28 g/mol | |
Source | PubChem | |
URL | https://pubchem.ncbi.nlm.nih.gov | |
Description | Data deposited in or computed by PubChem | |
CAS No. |
15869-94-0 | |
Record name | 3,6-Dimethyloctane | |
Source | CAS Common Chemistry | |
URL | https://commonchemistry.cas.org/detail?cas_rn=15869-94-0 | |
Description | CAS Common Chemistry is an open community resource for accessing chemical information. Nearly 500,000 chemical substances from CAS REGISTRY cover areas of community interest, including common and frequently regulated chemicals, and those relevant to high school and undergraduate chemistry classes. This chemical information, curated by our expert scientists, is provided in alignment with our mission as a division of the American Chemical Society. | |
Explanation | The data from CAS Common Chemistry is provided under a CC-BY-NC 4.0 license, unless otherwise stated. | |
Record name | Octane, 3,6-dimethyl- | |
Source | ChemIDplus | |
URL | https://pubchem.ncbi.nlm.nih.gov/substance/?source=chemidplus&sourceid=0015869940 | |
Description | ChemIDplus is a free, web search system that provides access to the structure and nomenclature authority files used for the identification of chemical substances cited in National Library of Medicine (NLM) databases, including the TOXNET system. | |
Record name | Octane, 3,6-dimethyl- | |
Source | EPA Chemicals under the TSCA | |
URL | https://www.epa.gov/chemicals-under-tsca | |
Description | EPA Chemicals under the Toxic Substances Control Act (TSCA) collection contains information on chemicals and their regulations under TSCA, including non-confidential content from the TSCA Chemical Substance Inventory and Chemical Data Reporting. | |
Record name | Octane, 3,6-dimethyl- | |
Source | EPA DSSTox | |
URL | https://comptox.epa.gov/dashboard/DTXSID50871247 | |
Description | DSSTox provides a high quality public chemistry resource for supporting improved predictive toxicology. | |
Record name | Octane, 3,6-dimethyl- | |
Source | European Chemicals Agency (ECHA) | |
URL | https://echa.europa.eu/information-on-chemicals | |
Description | The European Chemicals Agency (ECHA) is an agency of the European Union which is the driving force among regulatory authorities in implementing the EU's groundbreaking chemicals legislation for the benefit of human health and the environment as well as for innovation and competitiveness. | |
Explanation | Use of the information, documents and data from the ECHA website is subject to the terms and conditions of this Legal Notice, and subject to other binding limitations provided for under applicable law, the information, documents and data made available on the ECHA website may be reproduced, distributed and/or used, totally or in part, for non-commercial purposes provided that ECHA is acknowledged as the source: "Source: European Chemicals Agency, http://echa.europa.eu/". Such acknowledgement must be included in each copy of the material. ECHA permits and encourages organisations and individuals to create links to the ECHA website under the following cumulative conditions: Links can only be made to webpages that provide a link to the Legal Notice page. | |
Retrosynthesis Analysis
AI-Powered Synthesis Planning: Our tool employs the Template_relevance Pistachio, Template_relevance Bkms_metabolic, Template_relevance Pistachio_ringbreaker, Template_relevance Reaxys, Template_relevance Reaxys_biocatalysis model, leveraging a vast database of chemical reactions to predict feasible synthetic routes.
One-Step Synthesis Focus: Specifically designed for one-step synthesis, it provides concise and direct routes for your target compounds, streamlining the synthesis process.
Accurate Predictions: Utilizing the extensive PISTACHIO, BKMS_METABOLIC, PISTACHIO_RINGBREAKER, REAXYS, REAXYS_BIOCATALYSIS database, our tool offers high-accuracy predictions, reflecting the latest in chemical research and data.
Strategy Settings
Precursor scoring | Relevance Heuristic |
---|---|
Min. plausibility | 0.01 |
Model | Template_relevance |
Template Set | Pistachio/Bkms_metabolic/Pistachio_ringbreaker/Reaxys/Reaxys_biocatalysis |
Top-N result to add to graph | 6 |
Feasible Synthetic Routes
試験管内研究製品の免責事項と情報
BenchChemで提示されるすべての記事および製品情報は、情報提供を目的としています。BenchChemで購入可能な製品は、生体外研究のために特別に設計されています。生体外研究は、ラテン語の "in glass" に由来し、生物体の外で行われる実験を指します。これらの製品は医薬品または薬として分類されておらず、FDAから任何の医療状態、病気、または疾患の予防、治療、または治癒のために承認されていません。これらの製品を人間または動物に体内に導入する形態は、法律により厳格に禁止されています。これらのガイドラインに従うことは、研究と実験において法的および倫理的な基準の遵守を確実にするために重要です。