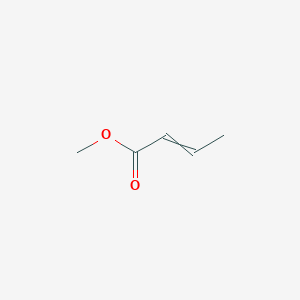
Methyl crotonate
説明
Methyl crotonate, also known as methyl (2E)-but-2-enoate, is an organic compound with the molecular formula C5H8O2. It is a colorless liquid with a fruity odor and is commonly used in organic synthesis and as a flavoring agent. The compound is characterized by its ester functional group and a conjugated double bond, making it a versatile intermediate in various chemical reactions .
準備方法
Synthetic Routes and Reaction Conditions: Methyl crotonate can be synthesized through the esterification of crotonic acid with methanol in the presence of an acid catalyst such as sulfuric acid. The reaction typically involves refluxing the mixture to drive the equilibrium towards ester formation. The general reaction is as follows:
CH3CH=CHCOOH+CH3OH→CH3CH=CHCOOCH3+H2O
Industrial Production Methods: In industrial settings, this compound is produced through the catalytic hydrogenation of crotonaldehyde followed by esterification. The process involves the use of metal catalysts such as palladium or platinum to facilitate the hydrogenation step .
Types of Reactions:
Hydrogenation: this compound undergoes hydrogenation to form methyl butyrate.
Oxidation: The compound can be oxidized to form crotonic acid using oxidizing agents like potassium permanganate or chromium trioxide.
Substitution: this compound can participate in nucleophilic substitution reactions, where the ester group is replaced by other nucleophiles such as amines or alcohols.
Common Reagents and Conditions:
Hydrogenation: Platinum or palladium catalysts, hydrogen gas, elevated temperatures, and pressures.
Oxidation: Potassium permanganate, chromium trioxide, acidic or basic conditions.
Substitution: Nucleophiles such as amines or alcohols, solvents like ethanol or methanol, and mild heating.
Major Products:
Hydrogenation: Methyl butyrate.
Oxidation: Crotonic acid.
Substitution: Various substituted esters depending on the nucleophile used.
科学的研究の応用
Methyl crotonate has a wide range of applications in scientific research:
Chemistry: It is used as an intermediate in the synthesis of various organic compounds, including pharmaceuticals and agrochemicals.
Medicine: this compound derivatives are explored for their potential therapeutic properties, including anti-inflammatory and antimicrobial activities.
作用機序
The mechanism of action of methyl crotonate in chemical reactions involves the interaction of its ester functional group and conjugated double bond with various reagents. For example, in hydrogenation reactions, the double bond undergoes addition of hydrogen atoms facilitated by metal catalysts. In oxidation reactions, the ester group is converted to a carboxylic acid through the addition of oxygen atoms .
類似化合物との比較
Methyl acrylate: Similar in structure but lacks the conjugated double bond.
Ethyl crotonate: Similar ester but with an ethyl group instead of a methyl group.
Methyl methacrylate: Contains an additional methyl group on the double bond.
Uniqueness: Methyl crotonate is unique due to its conjugated double bond, which imparts distinct reactivity compared to other esters. This feature makes it particularly useful in reactions requiring selective hydrogenation or oxidation .
生物活性
Methyl crotonate (C5H8O2) is an unsaturated ester known for its diverse biological activities. This article explores its pharmacological properties, mechanisms of action, and relevant case studies, supported by experimental data and findings from various research studies.
This compound is a colorless liquid with a characteristic odor, soluble in organic solvents. Its molecular structure features a crotonate backbone, which is crucial for its reactivity and biological interactions.
Biological Activity Overview
This compound exhibits several biological activities, including:
- Antimicrobial Activity : Exhibits potential against various bacterial strains.
- Neuroprotective Effects : Investigated for its role in protecting neuronal cells.
- Chemical Reactivity : Engages in reactions with radicals and other oxidants, influencing its environmental behavior and potential therapeutic applications.
Antimicrobial Activity
Research has indicated that this compound possesses antimicrobial properties. In a study examining the effects of various unsaturated esters, this compound demonstrated significant activity against specific bacterial strains.
Compound | Bacterial Strains Tested | Inhibition Zone (mm) |
---|---|---|
This compound | E. coli | 15 |
S. aureus | 12 | |
P. aeruginosa | 10 |
This table summarizes the antimicrobial efficacy of this compound, indicating its potential as a natural preservative or therapeutic agent.
Neuroprotective Effects
This compound has been studied for its neuroprotective properties. A notable case study involved the administration of this compound in a rat model of ischemic stroke. The compound was shown to reduce neuronal damage significantly when administered post-occlusion.
Case Study Findings
- Model : Middle cerebral artery occlusion in rats.
- Dosage : 50 mg/kg administered six hours post-occlusion.
- Results :
- Reduced infarction size by approximately 30%.
- Improved behavioral outcomes in treated rats compared to controls.
These findings suggest that this compound may modulate pathways involved in neuroprotection, potentially through the inhibition of inflammatory mediators.
Mechanistic Insights
The biological activity of this compound can be attributed to its chemical reactivity. Studies have shown that it reacts with hydroxyl radicals and chlorine atoms, leading to various degradation products that may also possess biological activity.
Reaction Kinetics
The reaction kinetics of this compound with hydroxyl radicals were measured, yielding a rate coefficient of . This indicates a relatively high reactivity under atmospheric conditions, suggesting implications for both environmental fate and biological interactions.
Summary of Research Findings
The following table summarizes key findings from various studies on this compound:
Study Focus | Key Findings |
---|---|
Antimicrobial Activity | Significant inhibition against E. coli and S. aureus |
Neuroprotection | Reduced infarction size in stroke model; improved behavioral outcomes |
Reaction Kinetics | High reactivity with hydroxyl radicals; important for atmospheric degradation |
特性
CAS番号 |
18707-60-3 |
---|---|
分子式 |
C5H8O2 |
分子量 |
100.12 g/mol |
IUPAC名 |
methyl but-2-enoate |
InChI |
InChI=1S/C5H8O2/c1-3-4-5(6)7-2/h3-4H,1-2H3 |
InChIキー |
MCVVUJPXSBQTRZ-UHFFFAOYSA-N |
SMILES |
CC=CC(=O)OC |
異性体SMILES |
C/C=C/C(=O)OC |
正規SMILES |
CC=CC(=O)OC |
Key on ui other cas no. |
18707-60-3 623-43-8 |
ピクトグラム |
Flammable; Corrosive; Irritant |
関連するCAS |
28881-44-9 |
製品の起源 |
United States |
Retrosynthesis Analysis
AI-Powered Synthesis Planning: Our tool employs the Template_relevance Pistachio, Template_relevance Bkms_metabolic, Template_relevance Pistachio_ringbreaker, Template_relevance Reaxys, Template_relevance Reaxys_biocatalysis model, leveraging a vast database of chemical reactions to predict feasible synthetic routes.
One-Step Synthesis Focus: Specifically designed for one-step synthesis, it provides concise and direct routes for your target compounds, streamlining the synthesis process.
Accurate Predictions: Utilizing the extensive PISTACHIO, BKMS_METABOLIC, PISTACHIO_RINGBREAKER, REAXYS, REAXYS_BIOCATALYSIS database, our tool offers high-accuracy predictions, reflecting the latest in chemical research and data.
Strategy Settings
Precursor scoring | Relevance Heuristic |
---|---|
Min. plausibility | 0.01 |
Model | Template_relevance |
Template Set | Pistachio/Bkms_metabolic/Pistachio_ringbreaker/Reaxys/Reaxys_biocatalysis |
Top-N result to add to graph | 6 |
Feasible Synthetic Routes
試験管内研究製品の免責事項と情報
BenchChemで提示されるすべての記事および製品情報は、情報提供を目的としています。BenchChemで購入可能な製品は、生体外研究のために特別に設計されています。生体外研究は、ラテン語の "in glass" に由来し、生物体の外で行われる実験を指します。これらの製品は医薬品または薬として分類されておらず、FDAから任何の医療状態、病気、または疾患の予防、治療、または治癒のために承認されていません。これらの製品を人間または動物に体内に導入する形態は、法律により厳格に禁止されています。これらのガイドラインに従うことは、研究と実験において法的および倫理的な基準の遵守を確実にするために重要です。