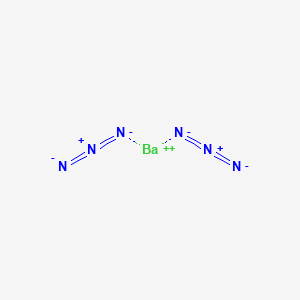
Barium azide (Ba(N3)2)
- 専門家チームからの見積もりを受け取るには、QUICK INQUIRYをクリックしてください。
- 品質商品を競争力のある価格で提供し、研究に集中できます。
説明
Barium azide is an inorganic azide with the formula Ba(N3)2 . It is a barium salt of hydrazoic acid. Like most azides, it is explosive and is less sensitive to mechanical shock than lead (II) azide .
Synthesis Analysis
Barium azide may be prepared by reacting sodium azide with a soluble barium salt . Care should be taken to prevent large crystals from forming in the solution as barium azide crystals will explode if subjected to friction/shock or if fully dried .Molecular Structure Analysis
Barium azide crystallizes in the space group P 2 1 / m . Each barium ion is surrounded by nine nitrogen ions . The azide ion is linear and nearly symmetrical .Chemical Reactions Analysis
Barium azide can be used to make azides of lithium, magnesium, potassium, rubidium, sodium, and zinc via substitution with their respective sulfates . .Physical and Chemical Properties Analysis
Barium azide is a white crystalline solid . It is odorless and has a density of 2.936 g/cm3 . It has a melting point of 126 °C and a boiling point of 160 °C . It is soluble in water and slightly soluble in ethanol .科学的研究の応用
Vibrational Spectra and Asymmetry in Crystals
Ba(N₃)₂ exhibits unique properties in its vibrational spectra, particularly in the symmetric stretching region. Studies like those conducted by Iqbal (1974) have shown interesting anisotropy in Raman and infrared peaks, relating to the localization of asymmetric azide ions (Iqbal, 1974).
Electron Spin Resonance (ESR) in Irradiated Crystals
Research by Marinkas and Bartram (1968) and further studies by Marinkas (1970) delve into the effects of ultraviolet irradiation on single crystals of Ba(N₃)₂. These studies have helped in understanding the ESR spectrum and the orientation of azide ions in the Ba(N₃)₂ lattice (Marinkas & Bartram, 1968), (Marinkas, 1970).
Structural Analysis of Ba(N₃)₂ Compounds
The crystal structure of Ba(N₃)₂ and its variants like BaN₃Cl have been the subject of studies like those by Blaschkowski et al. (2008), who analyzed their synthesis, crystal structure, vibrational spectra, and thermal decomposition. These studies contribute significantly to our understanding of the structural characteristics of Ba(N₃)₂-based compounds (Blaschkowski et al., 2008).
Azide Complexes of Barium
Research on azide complexes of barium, such as those by Brown et al. (2008), has led to the synthesis and characterization of novel structures. These studies offer insights into the coordination modes and the nature of metal-azide bonds in these complexes (Brown et al., 2008).
Decomposition of Metallic Azides
Understanding the decomposition process of metallic azides, including Ba(N₃)₂, has been a key area of research. Theoretical explanations for phenomena observed during decomposition by heat or irradiation have been explored in studies like those by Mott (1939) (Mott, 1939).
Identification of Radicals in Irradiated Azides
Investigations into the identification of radicals like N₃ or N₃²- in irradiated azides have been carried out, contributing to our understanding of the chemical changes in Ba(N₃)₂ under irradiation. Studies like those by Claxton, Overill, and Symons (1973) have used electron spin resonance spectroscopy and ab initio calculations for these purposes (Claxton, Overill, & Symons, 1973).
作用機序
Safety and Hazards
特性
IUPAC Name |
barium(2+);diazide |
Source
|
---|---|---|
Details | Computed by Lexichem TK 2.7.0 (PubChem release 2021.05.07) | |
Source | PubChem | |
URL | https://pubchem.ncbi.nlm.nih.gov | |
Description | Data deposited in or computed by PubChem | |
InChI |
InChI=1S/Ba.2N3/c;2*1-3-2/q+2;2*-1 |
Source
|
Details | Computed by InChI 1.0.6 (PubChem release 2021.05.07) | |
Source | PubChem | |
URL | https://pubchem.ncbi.nlm.nih.gov | |
Description | Data deposited in or computed by PubChem | |
InChI Key |
UUXFWHMUNNXFHD-UHFFFAOYSA-N |
Source
|
Details | Computed by InChI 1.0.6 (PubChem release 2021.05.07) | |
Source | PubChem | |
URL | https://pubchem.ncbi.nlm.nih.gov | |
Description | Data deposited in or computed by PubChem | |
Canonical SMILES |
[N-]=[N+]=[N-].[N-]=[N+]=[N-].[Ba+2] |
Source
|
Details | Computed by OEChem 2.3.0 (PubChem release 2021.05.07) | |
Source | PubChem | |
URL | https://pubchem.ncbi.nlm.nih.gov | |
Description | Data deposited in or computed by PubChem | |
Molecular Formula |
BaN6 |
Source
|
Details | Computed by PubChem 2.1 (PubChem release 2021.05.07) | |
Source | PubChem | |
URL | https://pubchem.ncbi.nlm.nih.gov | |
Description | Data deposited in or computed by PubChem | |
Molecular Weight |
221.37 g/mol |
Source
|
Details | Computed by PubChem 2.1 (PubChem release 2021.05.07) | |
Source | PubChem | |
URL | https://pubchem.ncbi.nlm.nih.gov | |
Description | Data deposited in or computed by PubChem | |
CAS No. |
18810-58-7 |
Source
|
Record name | Barium azide (Ba(N3)2) | |
Source | CAS Common Chemistry | |
URL | https://commonchemistry.cas.org/detail?cas_rn=18810-58-7 | |
Description | CAS Common Chemistry is an open community resource for accessing chemical information. Nearly 500,000 chemical substances from CAS REGISTRY cover areas of community interest, including common and frequently regulated chemicals, and those relevant to high school and undergraduate chemistry classes. This chemical information, curated by our expert scientists, is provided in alignment with our mission as a division of the American Chemical Society. | |
Explanation | The data from CAS Common Chemistry is provided under a CC-BY-NC 4.0 license, unless otherwise stated. | |
Retrosynthesis Analysis
AI-Powered Synthesis Planning: Our tool employs the Template_relevance Pistachio, Template_relevance Bkms_metabolic, Template_relevance Pistachio_ringbreaker, Template_relevance Reaxys, Template_relevance Reaxys_biocatalysis model, leveraging a vast database of chemical reactions to predict feasible synthetic routes.
One-Step Synthesis Focus: Specifically designed for one-step synthesis, it provides concise and direct routes for your target compounds, streamlining the synthesis process.
Accurate Predictions: Utilizing the extensive PISTACHIO, BKMS_METABOLIC, PISTACHIO_RINGBREAKER, REAXYS, REAXYS_BIOCATALYSIS database, our tool offers high-accuracy predictions, reflecting the latest in chemical research and data.
Strategy Settings
Precursor scoring | Relevance Heuristic |
---|---|
Min. plausibility | 0.01 |
Model | Template_relevance |
Template Set | Pistachio/Bkms_metabolic/Pistachio_ringbreaker/Reaxys/Reaxys_biocatalysis |
Top-N result to add to graph | 6 |
Feasible Synthetic Routes
試験管内研究製品の免責事項と情報
BenchChemで提示されるすべての記事および製品情報は、情報提供を目的としています。BenchChemで購入可能な製品は、生体外研究のために特別に設計されています。生体外研究は、ラテン語の "in glass" に由来し、生物体の外で行われる実験を指します。これらの製品は医薬品または薬として分類されておらず、FDAから任何の医療状態、病気、または疾患の予防、治療、または治癒のために承認されていません。これらの製品を人間または動物に体内に導入する形態は、法律により厳格に禁止されています。これらのガイドラインに従うことは、研究と実験において法的および倫理的な基準の遵守を確実にするために重要です。