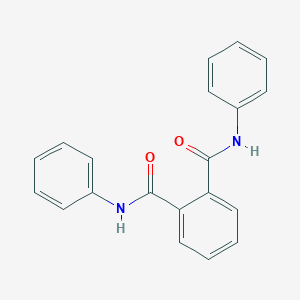
N,N'-Diphenylphthaldiamide
説明
N,N'-Diphenylphthaldiamide (DPPD) is an important organic compound used in a variety of scientific and industrial applications. It is a colorless, crystalline solid that is soluble in organic solvents and has a molecular weight of 250.89 g/mol. DPPD has been studied for its potential use in various fields, such as synthetic organic chemistry, analytical chemistry, and biochemistry. In particular, it is used as a reagent in the synthesis of complex organic molecules and as a catalyst in the synthesis of polymers. Additionally, it has been studied for its potential applications in biochemistry and drug development.
科学的研究の応用
Phosphatide Blockers
Phthalanilides were first described by Hirt and Berchtold in 1962 as “phosphatide blockers”. They used a simple biophysical model where lecithin dissolved in carbon tetrachloride promotes the transport of cationic dyes from an aqueous solution into the lipid phase .
Antineoplastic Agents
Phthalanilides have been shown to have activity against experimental neoplasms, leading to the synthesis of a large number of structurally related compounds . This suggests their potential use as antineoplastic agents.
Immunosuppressive Agents
The same properties that make phthalanilides effective as antineoplastic agents may also lend them to use as immunosuppressive agents . This could potentially be useful in treating autoimmune diseases or in preventing organ transplant rejection.
Lipid Complex Research
Phthalanilides have been used in research into lipid complexes. Their ability to inhibit dye transfer into a lipid phase has been used to study the properties of these complexes .
Mechanisms of Action in Leukemia
In lines of leukemias P815 and P388, resistance has been developed to three Wander derivatives of different structures. The lack of cross-resistance between certain of these derivatives suggests different mechanisms of action .
Chemotherapeutic Activity
Phthalanilides have been studied for their chemotherapeutic activity. Their rapid absorption by cells and inhibitory effect suggest potential use in chemotherapy .
作用機序
Target of Action
Phthalanilide, also known as N,N’-Diphenylphthaldiamide, primarily targets macromolecular lipids . These lipids are distributed ubiquitously in mammalian tissues, principally in the nucleoplasm of the cell . The extent of the complex formation between the phthalanilides and the macromolecular lipids relates to both therapy and toxicity of the drugs .
Mode of Action
Phthalanilide interacts with its targets by forming a complex with macromolecular lipids . This interaction inhibits the transfer of cationic dyes from an aqueous solution into the lipid phase . The compound maximally inhibits dye transfer when at least two strongly basic groups are connected by several coplanar rings and by carbonamide groups .
Biochemical Pathways
Phthalanilide affects the biochemical pathways related to the synthesis of DNA, protein, lipid, and RNA . At low intracellular concentrations, there is extensive inhibition of both DNA and protein but minimal chemotherapeutic response . Lipid and RNA synthesis is inhibited at therapeutic intracellular concentrations of the drug .
Pharmacokinetics
These properties play a crucial role in the bioavailability of the compound
Result of Action
The molecular and cellular effects of Phthalanilide’s action include a significant fall in cell electrophoretic mobility, suggesting that the drug forms an irreversible complex with cell surface components, thereby lowering their zeta potential . Moreover, the highest concentrations of Phthalanilide adversely affect the liver, kidney, and oculomotor muscles .
Action Environment
Environmental factors can influence the action, efficacy, and stability of Phthalanilide. It’s worth noting that environmental factors can significantly impact the behavior and effectiveness of many compounds .
特性
IUPAC Name |
1-N,2-N-diphenylbenzene-1,2-dicarboxamide | |
---|---|---|
Source | PubChem | |
URL | https://pubchem.ncbi.nlm.nih.gov | |
Description | Data deposited in or computed by PubChem | |
InChI |
InChI=1S/C20H16N2O2/c23-19(21-15-9-3-1-4-10-15)17-13-7-8-14-18(17)20(24)22-16-11-5-2-6-12-16/h1-14H,(H,21,23)(H,22,24) | |
Source | PubChem | |
URL | https://pubchem.ncbi.nlm.nih.gov | |
Description | Data deposited in or computed by PubChem | |
InChI Key |
ZYACJWRVLBPZNG-UHFFFAOYSA-N | |
Source | PubChem | |
URL | https://pubchem.ncbi.nlm.nih.gov | |
Description | Data deposited in or computed by PubChem | |
Canonical SMILES |
C1=CC=C(C=C1)NC(=O)C2=CC=CC=C2C(=O)NC3=CC=CC=C3 | |
Source | PubChem | |
URL | https://pubchem.ncbi.nlm.nih.gov | |
Description | Data deposited in or computed by PubChem | |
Molecular Formula |
C20H16N2O2 | |
Source | PubChem | |
URL | https://pubchem.ncbi.nlm.nih.gov | |
Description | Data deposited in or computed by PubChem | |
DSSTOX Substance ID |
DTXSID80167827 | |
Record name | N,N'-Diphenylphthaldiamide | |
Source | EPA DSSTox | |
URL | https://comptox.epa.gov/dashboard/DTXSID80167827 | |
Description | DSSTox provides a high quality public chemistry resource for supporting improved predictive toxicology. | |
Molecular Weight |
316.4 g/mol | |
Source | PubChem | |
URL | https://pubchem.ncbi.nlm.nih.gov | |
Description | Data deposited in or computed by PubChem | |
CAS RN |
16497-41-9 | |
Record name | N1,N2-Diphenyl-1,2-benzenedicarboxamide | |
Source | CAS Common Chemistry | |
URL | https://commonchemistry.cas.org/detail?cas_rn=16497-41-9 | |
Description | CAS Common Chemistry is an open community resource for accessing chemical information. Nearly 500,000 chemical substances from CAS REGISTRY cover areas of community interest, including common and frequently regulated chemicals, and those relevant to high school and undergraduate chemistry classes. This chemical information, curated by our expert scientists, is provided in alignment with our mission as a division of the American Chemical Society. | |
Explanation | The data from CAS Common Chemistry is provided under a CC-BY-NC 4.0 license, unless otherwise stated. | |
Record name | N,N'-Diphenylphthaldiamide | |
Source | ChemIDplus | |
URL | https://pubchem.ncbi.nlm.nih.gov/substance/?source=chemidplus&sourceid=0016497419 | |
Description | ChemIDplus is a free, web search system that provides access to the structure and nomenclature authority files used for the identification of chemical substances cited in National Library of Medicine (NLM) databases, including the TOXNET system. | |
Record name | N,N'-Diphenylphthaldiamide | |
Source | EPA DSSTox | |
URL | https://comptox.epa.gov/dashboard/DTXSID80167827 | |
Description | DSSTox provides a high quality public chemistry resource for supporting improved predictive toxicology. | |
Record name | N,N'-diphenylphthaldiamide | |
Source | European Chemicals Agency (ECHA) | |
URL | https://echa.europa.eu/substance-information/-/substanceinfo/100.036.861 | |
Description | The European Chemicals Agency (ECHA) is an agency of the European Union which is the driving force among regulatory authorities in implementing the EU's groundbreaking chemicals legislation for the benefit of human health and the environment as well as for innovation and competitiveness. | |
Explanation | Use of the information, documents and data from the ECHA website is subject to the terms and conditions of this Legal Notice, and subject to other binding limitations provided for under applicable law, the information, documents and data made available on the ECHA website may be reproduced, distributed and/or used, totally or in part, for non-commercial purposes provided that ECHA is acknowledged as the source: "Source: European Chemicals Agency, http://echa.europa.eu/". Such acknowledgement must be included in each copy of the material. ECHA permits and encourages organisations and individuals to create links to the ECHA website under the following cumulative conditions: Links can only be made to webpages that provide a link to the Legal Notice page. | |
Retrosynthesis Analysis
AI-Powered Synthesis Planning: Our tool employs the Template_relevance Pistachio, Template_relevance Bkms_metabolic, Template_relevance Pistachio_ringbreaker, Template_relevance Reaxys, Template_relevance Reaxys_biocatalysis model, leveraging a vast database of chemical reactions to predict feasible synthetic routes.
One-Step Synthesis Focus: Specifically designed for one-step synthesis, it provides concise and direct routes for your target compounds, streamlining the synthesis process.
Accurate Predictions: Utilizing the extensive PISTACHIO, BKMS_METABOLIC, PISTACHIO_RINGBREAKER, REAXYS, REAXYS_BIOCATALYSIS database, our tool offers high-accuracy predictions, reflecting the latest in chemical research and data.
Strategy Settings
Precursor scoring | Relevance Heuristic |
---|---|
Min. plausibility | 0.01 |
Model | Template_relevance |
Template Set | Pistachio/Bkms_metabolic/Pistachio_ringbreaker/Reaxys/Reaxys_biocatalysis |
Top-N result to add to graph | 6 |
Feasible Synthetic Routes
試験管内研究製品の免責事項と情報
BenchChemで提示されるすべての記事および製品情報は、情報提供を目的としています。BenchChemで購入可能な製品は、生体外研究のために特別に設計されています。生体外研究は、ラテン語の "in glass" に由来し、生物体の外で行われる実験を指します。これらの製品は医薬品または薬として分類されておらず、FDAから任何の医療状態、病気、または疾患の予防、治療、または治癒のために承認されていません。これらの製品を人間または動物に体内に導入する形態は、法律により厳格に禁止されています。これらのガイドラインに従うことは、研究と実験において法的および倫理的な基準の遵守を確実にするために重要です。