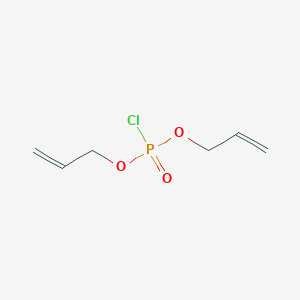
Diallyl chlorophosphate
説明
Diallyl chlorophosphate is an organophosphorus compound with the molecular formula C6H10ClO3P. It is a colorless liquid known for its use in organic synthesis, particularly in the formation of phosphates and phosphoramidates. The compound is characterized by the presence of two allyl groups attached to a phosphorus atom, which is also bonded to a chlorine atom and an oxygen atom.
準備方法
Synthetic Routes and Reaction Conditions: Diallyl chlorophosphate can be synthesized through the reaction of phosphorus trichloride with allyl alcohol in the presence of a base. The reaction typically proceeds as follows: [ \text{PCl}_3 + 3 \text{C}_3\text{H}_5\text{OH} \rightarrow \text{C}6\text{H}{10}\text{ClO}_3\text{P} + 2 \text{HCl} ]
Industrial Production Methods: In industrial settings, this compound is produced using continuous flow reactors to ensure efficient mixing and temperature control. The reaction is carried out under an inert atmosphere to prevent unwanted side reactions. The product is then purified through distillation or recrystallization.
Types of Reactions:
Substitution Reactions: this compound undergoes nucleophilic substitution reactions where the chlorine atom is replaced by various nucleophiles such as alcohols, amines, or thiols.
Oxidation Reactions: The compound can be oxidized to form diallyl phosphate, which is a useful intermediate in organic synthesis.
Common Reagents and Conditions:
Nucleophilic Substitution: Common reagents include alcohols (e.g., methanol, ethanol), amines (e.g., aniline), and thiols (e.g., thiophenol). The reactions are typically carried out in the presence of a base such as pyridine or triethylamine.
Oxidation: Oxidizing agents such as hydrogen peroxide or m-chloroperbenzoic acid are used under mild conditions to achieve the desired oxidation.
Major Products:
Diallyl Phosphate: Formed through oxidation.
Various Phosphoramidates and Phosphorothioates: Formed through substitution reactions with amines and thiols, respectively.
科学的研究の応用
Chemical Synthesis
Reagent in Organophosphorus Chemistry
Diallyl chlorophosphate serves as a crucial reagent in the synthesis of various organophosphorus compounds. It is particularly effective in creating phosphoramidates and phosphorothioates, which are essential in numerous chemical reactions and processes.
Synthesis of Dialkyl Chlorophosphates
Recent advancements have highlighted methods for synthesizing dialkyl chlorophosphates from dialkyl phosphites using this compound. This process is operationally simple and efficient, showcasing the compound's utility in organic synthesis .
Biological Applications
Enzyme Inhibition Studies
this compound is extensively used in biological research, particularly in studying enzyme inhibition. It has been identified as a potent inhibitor of cholinesterase enzymes, which play a critical role in neurotransmission. This property makes it valuable for developing pesticides and nerve agents.
Potential Therapeutic Uses
Research indicates that derivatives of this compound may act as prodrugs or be utilized in drug delivery systems. The ability to modulate biological pathways through enzyme inhibition positions this compound as a candidate for therapeutic applications in treating diseases influenced by cholinergic signaling.
Industrial Applications
Production of Specialty Chemicals
In industrial settings, this compound is employed in producing flame retardants and plasticizers. Its chemical properties allow it to enhance the performance and safety of various materials, making it an important component in manufacturing processes.
Case Study 1: Cholinesterase Inhibition
A study demonstrated that this compound effectively inhibited cholinesterase activity in vitro, leading to increased acetylcholine levels. This mechanism was crucial for understanding its potential applications in developing new pesticides that target cholinergic pathways.
Case Study 2: Synthesis of Phosphoramidates
In another investigation, researchers utilized this compound to synthesize phosphoramidates with high yields. The study highlighted the compound's efficiency as a reagent, paving the way for further exploration of its applications in pharmaceutical chemistry .
Comparative Data Table
作用機序
The mechanism of action of diallyl chlorophosphate involves the formation of a covalent bond between the phosphorus atom and the nucleophile. This process typically proceeds through a nucleophilic attack on the phosphorus atom, followed by the displacement of the chlorine atom. The resulting product depends on the nature of the nucleophile and the reaction conditions.
Molecular Targets and Pathways:
Enzyme Inhibition: this compound and its derivatives can inhibit cholinesterase enzymes by forming a covalent bond with the active site serine residue, leading to the accumulation of acetylcholine and subsequent overstimulation of cholinergic receptors.
Signal Transduction Pathways: The compound can modulate various signal transduction pathways by interacting with phosphorylated proteins and enzymes.
類似化合物との比較
Diethyl Chlorophosphate: Similar in structure but with ethyl groups instead of allyl groups.
Dimethyl Chlorophosphate: Contains methyl groups instead of allyl groups.
Dibutyl Chlorophosphate: Contains butyl groups instead of allyl groups.
Uniqueness: Diallyl chlorophosphate is unique due to the presence of allyl groups, which provide additional reactivity and versatility in organic synthesis. The allyl groups can participate in various reactions such as polymerization and cross-coupling, making this compound a valuable reagent in the synthesis of complex molecules.
生物活性
Diallyl chlorophosphate (DACP) is an organophosphorus compound that has garnered attention for its potential biological activities, particularly in the fields of pharmacology and toxicology. This article reviews the biological activity of DACP, focusing on its mechanisms of action, effects on cellular processes, and implications for health and disease.
This compound is characterized by the presence of two allyl groups attached to a phosphorus atom, along with a chlorophosphate functional group. It is typically synthesized through the reaction of diallyl phosphite with chlorinating agents such as carbon tetrachloride . The compound appears as a colorless to pale yellow liquid and exhibits reactivity due to the electrophilic nature of the phosphorus atom, making it a useful intermediate in organic synthesis.
1. Anticancer Properties
Research has indicated that DACP may exhibit anticancer properties similar to other diallyl compounds, such as diallyl disulfide (DADS). DADS has been shown to induce apoptosis in various cancer cell lines through multiple mechanisms, including:
- DNA Damage Induction : DADS promotes DNA damage by impairing nucleotide synthesis pathways, which may also be relevant for DACP .
- Inhibition of Cell Proliferation : Studies suggest that DACP could inhibit cell growth through mechanisms akin to those observed with DADS, potentially affecting signaling pathways involved in cell cycle regulation.
- Modulation of Apoptotic Pathways : Diallyl compounds can enhance apoptotic signaling by regulating proteins such as Bcl-2 and Bax, leading to increased apoptosis in cancer cells .
2. Neurotoxicity and Enzyme Inhibition
DACP's biological activity is not limited to anticancer effects; it also exhibits neurotoxic properties. Organophosphorus compounds are known inhibitors of acetylcholinesterase (AChE), which can lead to neurotoxic effects. The inhibition of AChE results in the accumulation of acetylcholine at synapses, causing overstimulation of cholinergic receptors and subsequent neurotoxicity.
Case Study 2: Neurotoxic Effects
Research into organophosphorus compounds has demonstrated their potential neurotoxic effects. A study highlighted that exposure to DACP resulted in significant inhibition of AChE activity in vitro, leading to increased levels of acetylcholine and subsequent neurotoxic symptoms in animal models. This finding underscores the dual nature of DACP's biological activity—beneficial in cancer treatment but potentially harmful as a neurotoxin.
特性
IUPAC Name |
3-[chloro(prop-2-enoxy)phosphoryl]oxyprop-1-ene | |
---|---|---|
Source | PubChem | |
URL | https://pubchem.ncbi.nlm.nih.gov | |
Description | Data deposited in or computed by PubChem | |
InChI |
InChI=1S/C6H10ClO3P/c1-3-5-9-11(7,8)10-6-4-2/h3-4H,1-2,5-6H2 | |
Source | PubChem | |
URL | https://pubchem.ncbi.nlm.nih.gov | |
Description | Data deposited in or computed by PubChem | |
InChI Key |
LEWQIRKQOJDHEC-UHFFFAOYSA-N | |
Source | PubChem | |
URL | https://pubchem.ncbi.nlm.nih.gov | |
Description | Data deposited in or computed by PubChem | |
Canonical SMILES |
C=CCOP(=O)(OCC=C)Cl | |
Source | PubChem | |
URL | https://pubchem.ncbi.nlm.nih.gov | |
Description | Data deposited in or computed by PubChem | |
Molecular Formula |
C6H10ClO3P | |
Source | PubChem | |
URL | https://pubchem.ncbi.nlm.nih.gov | |
Description | Data deposited in or computed by PubChem | |
DSSTOX Substance ID |
DTXSID70400513 | |
Record name | Diallyl chlorophosphate | |
Source | EPA DSSTox | |
URL | https://comptox.epa.gov/dashboard/DTXSID70400513 | |
Description | DSSTox provides a high quality public chemistry resource for supporting improved predictive toxicology. | |
Molecular Weight |
196.57 g/mol | |
Source | PubChem | |
URL | https://pubchem.ncbi.nlm.nih.gov | |
Description | Data deposited in or computed by PubChem | |
CAS No. |
16383-57-6 | |
Record name | Di-2-propen-1-yl phosphorochloridate | |
Source | CAS Common Chemistry | |
URL | https://commonchemistry.cas.org/detail?cas_rn=16383-57-6 | |
Description | CAS Common Chemistry is an open community resource for accessing chemical information. Nearly 500,000 chemical substances from CAS REGISTRY cover areas of community interest, including common and frequently regulated chemicals, and those relevant to high school and undergraduate chemistry classes. This chemical information, curated by our expert scientists, is provided in alignment with our mission as a division of the American Chemical Society. | |
Explanation | The data from CAS Common Chemistry is provided under a CC-BY-NC 4.0 license, unless otherwise stated. | |
Record name | Diallyl chlorophosphate | |
Source | EPA DSSTox | |
URL | https://comptox.epa.gov/dashboard/DTXSID70400513 | |
Description | DSSTox provides a high quality public chemistry resource for supporting improved predictive toxicology. | |
Retrosynthesis Analysis
AI-Powered Synthesis Planning: Our tool employs the Template_relevance Pistachio, Template_relevance Bkms_metabolic, Template_relevance Pistachio_ringbreaker, Template_relevance Reaxys, Template_relevance Reaxys_biocatalysis model, leveraging a vast database of chemical reactions to predict feasible synthetic routes.
One-Step Synthesis Focus: Specifically designed for one-step synthesis, it provides concise and direct routes for your target compounds, streamlining the synthesis process.
Accurate Predictions: Utilizing the extensive PISTACHIO, BKMS_METABOLIC, PISTACHIO_RINGBREAKER, REAXYS, REAXYS_BIOCATALYSIS database, our tool offers high-accuracy predictions, reflecting the latest in chemical research and data.
Strategy Settings
Precursor scoring | Relevance Heuristic |
---|---|
Min. plausibility | 0.01 |
Model | Template_relevance |
Template Set | Pistachio/Bkms_metabolic/Pistachio_ringbreaker/Reaxys/Reaxys_biocatalysis |
Top-N result to add to graph | 6 |
Feasible Synthetic Routes
試験管内研究製品の免責事項と情報
BenchChemで提示されるすべての記事および製品情報は、情報提供を目的としています。BenchChemで購入可能な製品は、生体外研究のために特別に設計されています。生体外研究は、ラテン語の "in glass" に由来し、生物体の外で行われる実験を指します。これらの製品は医薬品または薬として分類されておらず、FDAから任何の医療状態、病気、または疾患の予防、治療、または治癒のために承認されていません。これらの製品を人間または動物に体内に導入する形態は、法律により厳格に禁止されています。これらのガイドラインに従うことは、研究と実験において法的および倫理的な基準の遵守を確実にするために重要です。