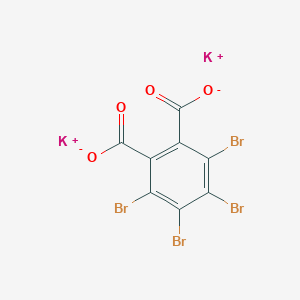
Dipotassium 3,4,5,6-tetrabromophthalate
カタログ番号 B095589
CAS番号:
18824-74-3
分子量: 557.89 g/mol
InChIキー: VQGCTIUJDSOQNU-UHFFFAOYSA-L
注意: 研究専用です。人間または獣医用ではありません。
説明
Dipotassium 3,4,5,6-tetrabromophthalate is a mono-constituent substance of organometallic origin . Its IUPAC name is Dipotassium 3,4,5,6-tetrabromophthalate and it has a molecular formula of C8H2Br4O4.2K .
Molecular Structure Analysis
The molecular structure of Dipotassium 3,4,5,6-tetrabromophthalate is based on the InChI annotation from the IUCLID reference substances database . The molecular formula is C8H2Br4O4.2K .Safety and Hazards
特性
IUPAC Name |
dipotassium;3,4,5,6-tetrabromophthalate | |
---|---|---|
Source | PubChem | |
URL | https://pubchem.ncbi.nlm.nih.gov | |
Description | Data deposited in or computed by PubChem | |
InChI |
InChI=1S/C8H2Br4O4.2K/c9-3-1(7(13)14)2(8(15)16)4(10)6(12)5(3)11;;/h(H,13,14)(H,15,16);;/q;2*+1/p-2 | |
Source | PubChem | |
URL | https://pubchem.ncbi.nlm.nih.gov | |
Description | Data deposited in or computed by PubChem | |
InChI Key |
VQGCTIUJDSOQNU-UHFFFAOYSA-L | |
Source | PubChem | |
URL | https://pubchem.ncbi.nlm.nih.gov | |
Description | Data deposited in or computed by PubChem | |
Canonical SMILES |
C1(=C(C(=C(C(=C1Br)Br)Br)Br)C(=O)[O-])C(=O)[O-].[K+].[K+] | |
Source | PubChem | |
URL | https://pubchem.ncbi.nlm.nih.gov | |
Description | Data deposited in or computed by PubChem | |
Molecular Formula |
C8Br4K2O4 | |
Source | PubChem | |
URL | https://pubchem.ncbi.nlm.nih.gov | |
Description | Data deposited in or computed by PubChem | |
Related CAS |
13810-83-8 (Parent) | |
Record name | 1,2-Benzenedicarboxylic acid, 3,4,5,6-tetrabromo-, potassium salt (1:2) | |
Source | ChemIDplus | |
URL | https://pubchem.ncbi.nlm.nih.gov/substance/?source=chemidplus&sourceid=0018824743 | |
Description | ChemIDplus is a free, web search system that provides access to the structure and nomenclature authority files used for the identification of chemical substances cited in National Library of Medicine (NLM) databases, including the TOXNET system. | |
DSSTOX Substance ID |
DTXSID00889670 | |
Record name | 1,2-Benzenedicarboxylic acid, 3,4,5,6-tetrabromo-, potassium salt (1:2) | |
Source | EPA DSSTox | |
URL | https://comptox.epa.gov/dashboard/DTXSID00889670 | |
Description | DSSTox provides a high quality public chemistry resource for supporting improved predictive toxicology. | |
Molecular Weight |
557.89 g/mol | |
Source | PubChem | |
URL | https://pubchem.ncbi.nlm.nih.gov | |
Description | Data deposited in or computed by PubChem | |
Product Name |
Dipotassium 3,4,5,6-tetrabromophthalate | |
CAS RN |
18824-74-3 | |
Record name | 1,2-Benzenedicarboxylic acid, 3,4,5,6-tetrabromo-, potassium salt (1:2) | |
Source | ChemIDplus | |
URL | https://pubchem.ncbi.nlm.nih.gov/substance/?source=chemidplus&sourceid=0018824743 | |
Description | ChemIDplus is a free, web search system that provides access to the structure and nomenclature authority files used for the identification of chemical substances cited in National Library of Medicine (NLM) databases, including the TOXNET system. | |
Record name | 1,2-Benzenedicarboxylic acid, 3,4,5,6-tetrabromo-, potassium salt (1:2) | |
Source | EPA Chemicals under the TSCA | |
URL | https://www.epa.gov/chemicals-under-tsca | |
Description | EPA Chemicals under the Toxic Substances Control Act (TSCA) collection contains information on chemicals and their regulations under TSCA, including non-confidential content from the TSCA Chemical Substance Inventory and Chemical Data Reporting. | |
Record name | 1,2-Benzenedicarboxylic acid, 3,4,5,6-tetrabromo-, potassium salt (1:2) | |
Source | EPA DSSTox | |
URL | https://comptox.epa.gov/dashboard/DTXSID00889670 | |
Description | DSSTox provides a high quality public chemistry resource for supporting improved predictive toxicology. | |
Record name | Dipotassium 3,4,5,6-tetrabromophthalate | |
Source | European Chemicals Agency (ECHA) | |
URL | https://echa.europa.eu/substance-information/-/substanceinfo/100.038.716 | |
Description | The European Chemicals Agency (ECHA) is an agency of the European Union which is the driving force among regulatory authorities in implementing the EU's groundbreaking chemicals legislation for the benefit of human health and the environment as well as for innovation and competitiveness. | |
Explanation | Use of the information, documents and data from the ECHA website is subject to the terms and conditions of this Legal Notice, and subject to other binding limitations provided for under applicable law, the information, documents and data made available on the ECHA website may be reproduced, distributed and/or used, totally or in part, for non-commercial purposes provided that ECHA is acknowledged as the source: "Source: European Chemicals Agency, http://echa.europa.eu/". Such acknowledgement must be included in each copy of the material. ECHA permits and encourages organisations and individuals to create links to the ECHA website under the following cumulative conditions: Links can only be made to webpages that provide a link to the Legal Notice page. | |
Synthesis routes and methods
Procedure details
A stainless steel kettle equipped with good agitation, heating and cooling was charged with 100 lbs. of water, 20 lbs. of polyethylene glycol (M.W. 200), 21/2 lbs. of triethanolamine, 421/2 lbs. of a non-ionic emulsifying agent (alkoxylated aryl polymer) and 40 lbs. of sorbitol. After mixing wascomplete, 10 lbs. of an anionic dispersing agent (the sodium salt of a formaldehyde-naphthalane sulfonic acid condensate) was added and the mixture stirred until all these additions were dissolved. With continued stirring, 500 lbs. of tetrabromophthalic anhydride were then added and mixed until uniformily dispersed. 1211/2 lbs. of 45 percent KOH were then added. The reaction was exothermic. The mix was then heated to about 90°-95° C. and stirring continued at 90°-95° C. until a smooth soft uniform paste was formed. The mix was then cooled to 75° C. and 1631/2 lbs. of a 2.6 percent Xanthan gum solution wasadded, the mix stirred until uniform and water added as necessary to make 1000 lbs. of paste. The resultant product was a smooth soft uniform paste of 65-66 percent solids, pH 2-3. A 5 percent aqueous dispersion of this product also had a pH of 2-3. One percent of the paste added to boiling water gave an almost clear solution with a pH of 2-3.
[Compound]
Name
stainless steel
Quantity
0 (± 1) mol
Type
reactant
Reaction Step One
[Compound]
Name
Xanthan gum
Quantity
0 (± 1) mol
Type
reactant
Reaction Step Two
[Compound]
Name
resultant product
Quantity
0 (± 1) mol
Type
reactant
Reaction Step Three
[Compound]
Name
solids
Quantity
0 (± 1) mol
Type
reactant
Reaction Step Four
[Compound]
Name
polyethylene glycol
Quantity
0 (± 1) mol
Type
reactant
Reaction Step Eight
[Compound]
Name
alkoxylated aryl
Quantity
0 (± 1) mol
Type
reactant
Reaction Step Ten
Name
sorbitol
Quantity
0 (± 1) mol
Type
reactant
Reaction Step Eleven
[Compound]
Name
formaldehyde naphthalane sulfonic acid
Quantity
0 (± 1) mol
Type
reactant
Reaction Step Twelve
試験管内研究製品の免責事項と情報
BenchChemで提示されるすべての記事および製品情報は、情報提供を目的としています。BenchChemで購入可能な製品は、生体外研究のために特別に設計されています。生体外研究は、ラテン語の "in glass" に由来し、生物体の外で行われる実験を指します。これらの製品は医薬品または薬として分類されておらず、FDAから任何の医療状態、病気、または疾患の予防、治療、または治癒のために承認されていません。これらの製品を人間または動物に体内に導入する形態は、法律により厳格に禁止されています。これらのガイドラインに従うことは、研究と実験において法的および倫理的な基準の遵守を確実にするために重要です。