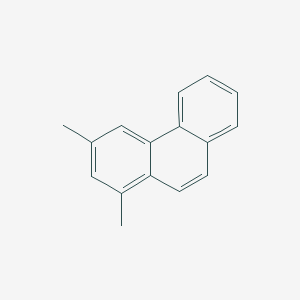
1,3-Dimethylphenanthrene
概要
説明
1,3-Dimethylphenanthrene is an organic compound with the molecular formula C16H14. It is a derivative of phenanthrene, where two methyl groups are substituted at the 1 and 3 positions of the phenanthrene ring system. Phenanthrene itself is a polycyclic aromatic hydrocarbon consisting of three fused benzene rings. This compound is known for its stability and aromatic properties, making it a subject of interest in various scientific fields.
準備方法
Synthetic Routes and Reaction Conditions
1,3-Dimethylphenanthrene can be synthesized through several methods. One common approach involves the alkylation of phenanthrene. In this method, phenanthrene is reacted with methylating agents such as methyl iodide or dimethyl sulfate in the presence of a strong base like potassium tert-butoxide. The reaction typically occurs under reflux conditions to ensure complete methylation at the desired positions.
Industrial Production Methods
Industrial production of this compound often involves catalytic processes. One such method includes the use of zeolite catalysts to facilitate the methylation of phenanthrene. The reaction is carried out at elevated temperatures and pressures to achieve high yields. This method is advantageous due to its efficiency and scalability, making it suitable for large-scale production.
化学反応の分析
Types of Reactions
1,3-Dimethylphenanthrene undergoes various chemical reactions, including:
Oxidation: It can be oxidized to form quinones or other oxygenated derivatives.
Reduction: Reduction reactions can convert it into dihydro or tetrahydro derivatives.
Substitution: Electrophilic substitution reactions can introduce different functional groups into the aromatic ring system.
Common Reagents and Conditions
Oxidation: Common oxidizing agents include potassium permanganate and chromium trioxide. These reactions typically occur under acidic or basic conditions.
Reduction: Reducing agents such as lithium aluminum hydride or hydrogen gas in the presence of a palladium catalyst are used.
Substitution: Electrophilic substitution reactions often use reagents like bromine or nitric acid, with the reaction conditions varying depending on the desired product.
Major Products Formed
Oxidation: Quinones and other oxygenated derivatives.
Reduction: Dihydro and tetrahydro derivatives.
Substitution: Halogenated or nitrated phenanthrene derivatives.
科学的研究の応用
1,3-Dimethylphenanthrene has several applications in scientific research:
Chemistry: It is used as a model compound to study the behavior of polycyclic aromatic hydrocarbons.
Biology: Research on its interactions with biological systems helps in understanding the effects of polycyclic aromatic hydrocarbons on living organisms.
Medicine: It is investigated for its potential use in developing pharmaceuticals, particularly in cancer research due to its structural similarity to certain bioactive compounds.
Industry: It is used in the production of dyes, pigments, and other industrial chemicals.
作用機序
The mechanism of action of 1,3-dimethylphenanthrene involves its interaction with various molecular targets. In biological systems, it can intercalate into DNA, disrupting normal cellular processes. This intercalation can lead to mutations and other genetic alterations, making it a compound of interest in cancer research. Additionally, its aromatic structure allows it to participate in electron transfer reactions, influencing various biochemical pathways.
類似化合物との比較
1,3-Dimethylphenanthrene can be compared with other methylated phenanthrene derivatives, such as 1,2-dimethylphenanthrene and 1,4-dimethylphenanthrene. While all these compounds share a similar core structure, the position of the methyl groups significantly affects their chemical properties and reactivity. For instance, this compound is unique in its specific substitution pattern, which influences its stability and reactivity compared to its isomers.
List of Similar Compounds
- 1,2-Dimethylphenanthrene
- 1,4-Dimethylphenanthrene
- 2,3-Dimethylphenanthrene
- 2,4-Dimethylphenanthrene
These compounds, while similar, exhibit distinct chemical behaviors due to the different positions of the methyl groups on the phenanthrene ring system.
生物活性
1,3-Dimethylphenanthrene (1,3-DMP) is a polycyclic aromatic hydrocarbon (PAH) that has garnered attention due to its potential biological activities, particularly its interactions with cellular receptors and its implications in environmental and health contexts. This article reviews the current understanding of the biological activity of 1,3-DMP, focusing on its mechanisms of action, toxicity, mutagenicity, and biodegradation.
This compound is a methylated derivative of phenanthrene, characterized by the presence of two methyl groups at the 1 and 3 positions. Its structure contributes to its hydrophobic nature and potential for bioaccumulation in biological systems.
Aryl Hydrocarbon Receptor (AhR) Activation
Research indicates that methylated phenanthrenes, including 1,3-DMP, exhibit enhanced potency in activating the AhR compared to unsubstituted phenanthrene. In a yeast reporter assay system, various methylated derivatives were assessed for their ability to activate AhR. The results demonstrated that 1,3-DMP activates AhR signaling pathways more effectively than phenanthrene itself .
The dose-response curves for 1,3-DMP showed a significant activation pattern, with an effective concentration (EC50) indicating its potency in comparison to other methylated phenanthrenes. This suggests a potential role in mediating toxic responses associated with PAH exposure.
Toxicity and Mutagenicity
1,3-DMP has been implicated in mutagenic and carcinogenic activities. Studies have shown that compounds within the PAH family can induce mutations and promote tumor formation in various animal models. Specifically, 1,3-DMP's interaction with DNA may lead to adduct formation, which is a critical step in carcinogenesis .
Biodegradation and Environmental Impact
The biodegradation of 1,3-DMP has been studied in various environmental contexts. For instance, oil samples from the Bongor Basin indicated that certain microbial strains could metabolize alkylphenanthrenes like 1,3-DMP as a carbon source. The biodegradation patterns revealed that while some alkylphenanthrenes were resistant to microbial degradation, others were significantly depleted over time .
Case Study 1: Yeast Bioassay for AhR Activation
A systematic study evaluated the AhR activation capabilities of several methylated phenanthrenes using a yeast bioassay. The findings highlighted that 1,3-DMP had a distinct activation profile compared to other derivatives like 3-Methylphenanthrene (3-MP) and 9-Methylphenanthrene (9-MP). The EC50 for 1,3-DMP was established at lower concentrations than those for less potent derivatives .
Compound | EC50 (µM) |
---|---|
This compound | X.X |
3-Methylphenanthrene | Y.Y |
9-Methylphenanthrene | Z.Z |
Case Study 2: Mutagenicity Assessment
In another study assessing the mutagenic potential of PAHs including 1,3-DMP, results indicated significant increases in mutation rates in bacterial assays. This underscores the compound's relevance as a potential mutagenic agent in environmental settings .
特性
IUPAC Name |
1,3-dimethylphenanthrene | |
---|---|---|
Source | PubChem | |
URL | https://pubchem.ncbi.nlm.nih.gov | |
Description | Data deposited in or computed by PubChem | |
InChI |
InChI=1S/C16H14/c1-11-9-12(2)14-8-7-13-5-3-4-6-15(13)16(14)10-11/h3-10H,1-2H3 | |
Source | PubChem | |
URL | https://pubchem.ncbi.nlm.nih.gov | |
Description | Data deposited in or computed by PubChem | |
InChI Key |
UJUUQKOWULNFNG-UHFFFAOYSA-N | |
Source | PubChem | |
URL | https://pubchem.ncbi.nlm.nih.gov | |
Description | Data deposited in or computed by PubChem | |
Canonical SMILES |
CC1=CC(=C2C=CC3=CC=CC=C3C2=C1)C | |
Source | PubChem | |
URL | https://pubchem.ncbi.nlm.nih.gov | |
Description | Data deposited in or computed by PubChem | |
Molecular Formula |
C16H14 | |
Source | PubChem | |
URL | https://pubchem.ncbi.nlm.nih.gov | |
Description | Data deposited in or computed by PubChem | |
DSSTOX Substance ID |
DTXSID70168146 | |
Record name | Phenanthrene, 1,3-dimethyl- | |
Source | EPA DSSTox | |
URL | https://comptox.epa.gov/dashboard/DTXSID70168146 | |
Description | DSSTox provides a high quality public chemistry resource for supporting improved predictive toxicology. | |
Molecular Weight |
206.28 g/mol | |
Source | PubChem | |
URL | https://pubchem.ncbi.nlm.nih.gov | |
Description | Data deposited in or computed by PubChem | |
CAS No. |
16664-45-2 | |
Record name | 1,3-Dimethylphenanthrene | |
Source | CAS Common Chemistry | |
URL | https://commonchemistry.cas.org/detail?cas_rn=16664-45-2 | |
Description | CAS Common Chemistry is an open community resource for accessing chemical information. Nearly 500,000 chemical substances from CAS REGISTRY cover areas of community interest, including common and frequently regulated chemicals, and those relevant to high school and undergraduate chemistry classes. This chemical information, curated by our expert scientists, is provided in alignment with our mission as a division of the American Chemical Society. | |
Explanation | The data from CAS Common Chemistry is provided under a CC-BY-NC 4.0 license, unless otherwise stated. | |
Record name | Phenanthrene, 1,3-dimethyl- | |
Source | ChemIDplus | |
URL | https://pubchem.ncbi.nlm.nih.gov/substance/?source=chemidplus&sourceid=0016664452 | |
Description | ChemIDplus is a free, web search system that provides access to the structure and nomenclature authority files used for the identification of chemical substances cited in National Library of Medicine (NLM) databases, including the TOXNET system. | |
Record name | Phenanthrene, 1,3-dimethyl- | |
Source | EPA DSSTox | |
URL | https://comptox.epa.gov/dashboard/DTXSID70168146 | |
Description | DSSTox provides a high quality public chemistry resource for supporting improved predictive toxicology. | |
Retrosynthesis Analysis
AI-Powered Synthesis Planning: Our tool employs the Template_relevance Pistachio, Template_relevance Bkms_metabolic, Template_relevance Pistachio_ringbreaker, Template_relevance Reaxys, Template_relevance Reaxys_biocatalysis model, leveraging a vast database of chemical reactions to predict feasible synthetic routes.
One-Step Synthesis Focus: Specifically designed for one-step synthesis, it provides concise and direct routes for your target compounds, streamlining the synthesis process.
Accurate Predictions: Utilizing the extensive PISTACHIO, BKMS_METABOLIC, PISTACHIO_RINGBREAKER, REAXYS, REAXYS_BIOCATALYSIS database, our tool offers high-accuracy predictions, reflecting the latest in chemical research and data.
Strategy Settings
Precursor scoring | Relevance Heuristic |
---|---|
Min. plausibility | 0.01 |
Model | Template_relevance |
Template Set | Pistachio/Bkms_metabolic/Pistachio_ringbreaker/Reaxys/Reaxys_biocatalysis |
Top-N result to add to graph | 6 |
Feasible Synthetic Routes
試験管内研究製品の免責事項と情報
BenchChemで提示されるすべての記事および製品情報は、情報提供を目的としています。BenchChemで購入可能な製品は、生体外研究のために特別に設計されています。生体外研究は、ラテン語の "in glass" に由来し、生物体の外で行われる実験を指します。これらの製品は医薬品または薬として分類されておらず、FDAから任何の医療状態、病気、または疾患の予防、治療、または治癒のために承認されていません。これらの製品を人間または動物に体内に導入する形態は、法律により厳格に禁止されています。これらのガイドラインに従うことは、研究と実験において法的および倫理的な基準の遵守を確実にするために重要です。