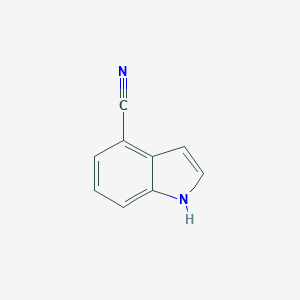
4-Cyanoindole
概要
説明
4-Cyanoindole (4-CI) is a substituted indole derivative characterized by a cyano group (-CN) at the 4-position of the indole ring. This modification significantly alters its electronic and photophysical properties compared to unsubstituted indole. Quantum chemical calculations (SCS-CC2/cc-pVTZ) confirm its planar geometry in both ground (S₀) and lowest excited singlet (S₁) states . The S₁ state exhibits 1Lα symmetry, marked by a large increase in permanent dipole moment from 6.31 D (S₀) to 8.92 D (S₁), a hallmark of 1Lα character . In the gas phase, 4-CI has an excited-state lifetime of 11.0 ns, which shortens to 5–6 ns in deuterated isotopologues (e.g., N-D, C(2)-D, C(3)-D) due to isotopic effects on non-radiative decay pathways . Its fluorescence quantum yield is 0.42 in isolation and 0.85 in aqueous solution, making it a robust fluorescent probe .
準備方法
Synthetic Routes and Reaction Conditions: 4-Cyanoindole can be synthesized through several methods. One common approach involves the reaction of indole with cyanogen bromide in the presence of a base. The reaction typically proceeds under mild conditions, yielding this compound as the primary product. Another method involves the palladium-catalyzed cyanation of 4-bromoindole using a cyanide source such as potassium cyanide or zinc cyanide.
Industrial Production Methods: In an industrial setting, the synthesis of this compound may involve large-scale reactions using optimized conditions to maximize yield and purity. The use of continuous flow reactors and advanced purification techniques, such as recrystallization and chromatography, ensures the efficient production of high-quality this compound.
化学反応の分析
Types of Reactions: 4-Cyanoindole undergoes various chemical reactions, including:
Oxidation: The cyano group can be oxidized to form carboxylic acids or amides.
Reduction: The cyano group can be reduced to form primary amines.
Substitution: The indole ring can undergo electrophilic substitution reactions, such as halogenation, nitration, and sulfonation.
Common Reagents and Conditions:
Oxidation: Reagents such as potassium permanganate or chromium trioxide are commonly used.
Reduction: Catalytic hydrogenation or the use of reducing agents like lithium aluminum hydride.
Substitution: Electrophilic reagents like bromine, nitric acid, or sulfuric acid.
Major Products Formed:
Oxidation: Carboxylic acids, amides.
Reduction: Primary amines.
Substitution: Halogenated, nitrated, or sulfonated indole derivatives.
科学的研究の応用
Fluorescent Probes in Biochemical Research
Fluorescent Nucleosides
4CIN has been developed as a highly efficient fluorescent nucleoside analogue. Its incorporation into DNA demonstrates remarkable fluorescence characteristics, with quantum yields exceeding 0.90 in aqueous environments. When integrated into duplex DNA, 4CIN exhibits a range of quantum yields (0.15–0.31), outperforming traditional fluorophores like 2-aminopurine (2APN) . This makes 4CIN an excellent candidate for probing DNA structures and dynamics.
Case Study: Fluorescence Quenching
Research has shown that the fluorescence-quencher pair formed by 4CIN and guanine can quantitatively assess binding affinities within DNA sequences. This application is crucial for understanding molecular interactions at the nucleic acid level .
Spectroscopic Applications
Biological Spectroscopy
The unique properties of this compound derivatives allow them to serve as effective labeling agents in biological spectroscopy. The close structural resemblance to natural amino acids minimizes perturbation to biological systems while enabling detailed studies through fluorescence-based techniques .
Case Study: Spectroscopic Utility
In studies involving unnatural amino acids like 4-cyanotryptophan (4CN-Trp), researchers have utilized these fluorophores to explore various biological processes, demonstrating their broad utility in microscopy and fluorescence resonance energy transfer (FRET) applications .
Structural Probes for DNA and RNA
Site-Specific IR Probing
The C≡N stretching vibration of 4CIN can be employed as a site-specific infrared (IR) probe, providing insights into the local hydration and electrostatic environments of DNA and RNA structures. The sensitivity of this vibrational frequency to solvent conditions enhances its applicability in studying biomolecular conformations .
Synthesis and Characterization
Synthesis Techniques
The synthesis of this compound derivatives is well-documented, allowing for the production of various analogues with tailored properties for specific applications. Recent research has focused on optimizing these synthesis protocols to improve yield and efficiency .
Compound | Quantum Yield | Application |
---|---|---|
This compound | >0.90 | Fluorescent probe for nucleic acids |
This compound-2'-deoxyribonucleoside (4CIN) | 0.15–0.31 | DNA structure probing, binding affinity assessment |
4-Cyanotryptophan | Variable | Biological spectroscopy and imaging |
作用機序
The mechanism of action of 4-cyanoindole and its derivatives often involves interaction with specific molecular targets, such as enzymes or receptors. The cyano group can participate in hydrogen bonding and other non-covalent interactions, influencing the binding affinity and specificity of the compound. In biological systems, this compound derivatives may inhibit enzyme activity or modulate receptor signaling pathways, leading to therapeutic effects.
類似化合物との比較
Comparison with Similar Compounds
Electronic Structure and Symmetry
The lowest excited singlet state (S₁) symmetry distinguishes 4-CI from its isomers:
Key Insight: The position of the cyano group dictates S₁ symmetry. 4- and 5-substitution favor 1Lα, while 3-substitution stabilizes 1Lβ due to differences in electron density redistribution .
Photophysical Properties
Table 1: Excited-State Lifetimes and Quantum Yields
Compound | Gas-Phase Lifetime (ns) | Lifetime in Solution (ns) | Quantum Yield (Isolated) | Quantum Yield (H₂O) |
---|---|---|---|---|
This compound | 11.0 | 4.7 (D₂O) | 0.42 | 0.85 |
3-Cyanoindole | 9.8 → 14.8 (D) | N/A | 0.43 | N/A |
5-Cyanoindole | N/A | N/A | 0.10 | N/A |
Indole | 16.4 → 17.2 (N-D) | <1.0 (polar solvents) | <0.1 | <0.1 |
Deuteration Effects :
- 4-CI’s lifetime shortens upon deuteration (e.g., N-D: 5.0 ns), whereas 3-CI’s lifetime increases (9.8 ns → 14.8 ns) .
- This contrast suggests differing non-radiative decay mechanisms: 4-CI’s decay is vibrationally coupled to N-H/C-H modes, while 3-CI’s involves torsional motion .
Dipole Moments and Transition Dipole Moments (TDM)
Table 2: Dipole Moment Changes and TDM Orientation
Key Observations :
- The TDM angle (relative to the indole pseudo-C₂ axis) is similar for 4-CI (+30.71°) and indole (+38.1°), indicating conserved transition alignment despite substitution .
- 3-CI’s negative Δμ and distinct TDM angle reflect its 1Lβ character, which localizes electron density away from the cyano group .
Solvent and Environmental Sensitivity
- 4-CI : Exhibits strong solvent-dependent fluorescence. In water (μ = 1.85 D), its quantum yield rises to 0.85 due to stabilization of the polar S₁ state, whereas in ethyl acetate (μ = 1.78 D), the yield drops .
- 5-CI: Lower quantum yield (0.10) suggests greater susceptibility to non-radiative decay, possibly due to enhanced spin-orbit coupling .
- 3-CI: Limited solubility data, but its 1Lβ state likely reduces polarity-driven solvent effects .
生物活性
4-Cyanoindole (4-CI) is a synthetic compound that has garnered attention for its unique biological properties, particularly as a fluorescent probe in biochemical research. This article explores the biological activity of this compound, focusing on its applications in DNA/RNA studies, fluorescence spectroscopy, and its potential as a therapeutic agent.
This compound is characterized by the chemical formula and a molecular weight of 158.16 g/mol. Its structure features a cyano group () attached to the indole ring, which enhances its spectroscopic properties.
Spectroscopic Properties
The incorporation of this compound into nucleosides has been shown to significantly improve their fluorescence characteristics:
- Fluorescence Quantum Yield : this compound exhibits a high quantum yield, making it an effective fluorescent probe. Studies indicate that it maintains superior spectroscopic properties compared to traditional nucleobase analogs like 2-aminopurine (2AP) when incorporated into DNA .
- Sensitivity to Environment : The C≡N stretching frequency of this compound is sensitive to its local environment, allowing it to act as a site-specific infrared probe for oligonucleotides .
1. Fluorescent Nucleosides
This compound has been synthesized into various nucleoside forms, including this compound-2'-deoxyribonucleoside (4CIN) and this compound-ribonucleoside (4CINr). These compounds are utilized in:
- DNA Hybridization Studies : The incorporation of 4CIN into DNA strands allows researchers to study hybridization processes with enhanced fluorescence detection capabilities .
- Enzymatic Kinetics : Assays involving DNA polymerases have demonstrated that 4CIN can be efficiently incorporated into DNA, providing insights into enzymatic mechanisms and kinetics .
2. Fluorescence Quenching Studies
The interaction between this compound and guanine has been explored as a model for understanding DNA-protein interactions:
- Fluorophore-Quencher Pairs : The fluorescence of this compound can be quenched by guanine, enabling quantitative assessments of binding affinities between single-stranded DNA and proteins such as bovine serum albumin (BSA) . This property is particularly useful for high-throughput screening of DNA-protein interactions.
3. Therapeutic Potential
Emerging studies suggest that derivatives of this compound may possess therapeutic properties due to their ability to modulate biological pathways:
- Antimicrobial Activity : Research indicates that indole derivatives can influence bacterial signaling pathways, potentially offering new avenues for antibiotic development .
Case Study 1: Fluorescence Lifetime Measurements
A study examined the fluorescence lifetime of various cyanoindoles in different solvents. It was found that this compound exhibited a longer fluorescence lifetime in aqueous environments compared to other derivatives, making it suitable for biological applications where water solubility is crucial .
Case Study 2: Binding Affinity Assays
In another investigation, fluorescence intensity measurements were used to determine the binding constant of a single-stranded DNA containing this compound to BSA. The results showed a significant increase in fluorescence upon binding, confirming the utility of this compound in studying protein-DNA interactions .
Data Tables
Property | Value |
---|---|
Molecular Formula | |
Molecular Weight | 158.16 g/mol |
Fluorescence Quantum Yield | High |
Solvent Dependence | Longer lifetime in water |
Application | Description |
---|---|
DNA Hybridization | Enhanced detection via fluorescence |
Enzymatic Kinetics | Assay for polymerase activity |
Protein Binding | Quantitative binding assays |
Q & A
Basic Research Questions
Q. What experimental methods are recommended for synthesizing 4-cyanoindole in academic settings?
Aqueous-phase synthesis using equimolar this compound and serine at controlled temperatures (e.g., 25–45°C) achieves high turnover frequencies (up to 1,000 turnovers) with minimal byproducts. HPLC analysis with 254-nm UV detection is critical for quantifying product formation and purity . Gram-scale preparations require iterative optimization of reaction conditions (pH, solvent polarity) to suppress side reactions.
Q. How is the fluorescence lifetime of this compound experimentally determined?
Time-correlated single-photon counting (TCSPC) is the gold standard. For gas-phase studies, rotational-resolved electronic Stark spectroscopy isolates Lorentzian contributions to fluorescence decay, yielding lifetimes (e.g., 11.0 ns for isolated this compound). In solution, TCSPC with ultrafast lasers (e.g., DeltaFlex Ultima) measures single-exponential decays, revealing solvent-dependent variations (e.g., 4.7 ns in D₂O vs. shorter lifetimes in ethyl acetate) .
Q. Which spectroscopic techniques are essential for characterizing this compound’s electronic structure?
Rotational-resolved fluorescence and electronic Stark spectroscopy determine transition dipole moment (TDM) angles (e.g., +38.1° for indole vs. +35.1° for 3-cyanoindole). Franck-Condon analysis of dispersed fluorescence (DF) spectra quantifies structural changes between ground (S₀) and excited (S₁) states, confirming planar geometries .
Advanced Research Questions
Q. How can contradictions in solvent-dependent fluorescence lifetimes be resolved?
Despite similar solvent dipole moments (e.g., H₂O: 1.85 D vs. ethyl acetate: 1.78 D), this compound exhibits shorter lifetimes in ethyl acetate. This contradicts the "polar solvent stabilizes ground state" hypothesis. Instead, solvent-solute coupling (e.g., H-bonding in water) modulates conical intersection (CI) accessibility, as proposed by Burghardt and Hynes. Validate this via temperature-dependent TCSPC and molecular dynamics simulations .
Q. What methodological challenges arise when determining TDM angles in substituted indoles?
Axis rotation Hamiltonian fitting (±± sign combinations) minimizes intensity deviations between experimental and simulated spectra. For this compound, TDM angles are referenced to the pseudo-C₂ axis of indole, requiring corrections for inertial axis shifts due to cyano substitution. Deuterated analogs (e.g., N(1)-D) confirm rotational directionality via y-angle reductions (30.71° → 28.71°) .
Q. How do isotopic substitutions (e.g., deuterium) alter excited-state dynamics?
Deuteration at N(1)-H increases gas-phase lifetimes (non-deuterated: 11.0 ns vs. N(1)-D: 5.0 ns), while C(2)-D and C(3)-D substitutions yield intermediate values (6.19 ns and 5.8 ns, respectively). This suggests site-specific vibronic coupling to high-frequency N-H stretching modes. Design isotope-editing experiments with site-selective deuteration to isolate mode-specific contributions .
Q. Why do computational models underestimate excited-state dipole moments in this compound?
Ab initio calculations often fail to account for solvent-induced charge redistribution. Experimental Stark spectroscopy reveals a 3.5 D increase in dipole moment upon excitation (S₀: 2.1 D → S₁: 5.6 D). Hybrid QM/MM simulations incorporating explicit solvent molecules improve agreement but require iterative refinement of solvent cavity parameters .
Q. What strategies reconcile discrepancies between fluorescence quantum yields (Φ) in isolated vs. solvated systems?
Isolated this compound has Φ = 0.42, but aqueous solutions show Φ = 0.85 due to suppressed non-radiative decay pathways. Compare with 5-cyanoindole (Φ = 0.10), where steric hindrance from the cyano group enhances internal conversion. Use transient absorption spectroscopy to quantify competing decay channels (e.g., intersystem crossing) .
特性
IUPAC Name |
1H-indole-4-carbonitrile | |
---|---|---|
Source | PubChem | |
URL | https://pubchem.ncbi.nlm.nih.gov | |
Description | Data deposited in or computed by PubChem | |
InChI |
InChI=1S/C9H6N2/c10-6-7-2-1-3-9-8(7)4-5-11-9/h1-5,11H | |
Source | PubChem | |
URL | https://pubchem.ncbi.nlm.nih.gov | |
Description | Data deposited in or computed by PubChem | |
InChI Key |
CEUFGDDOMXCXFW-UHFFFAOYSA-N | |
Source | PubChem | |
URL | https://pubchem.ncbi.nlm.nih.gov | |
Description | Data deposited in or computed by PubChem | |
Canonical SMILES |
C1=CC(=C2C=CNC2=C1)C#N | |
Source | PubChem | |
URL | https://pubchem.ncbi.nlm.nih.gov | |
Description | Data deposited in or computed by PubChem | |
Molecular Formula |
C9H6N2 | |
Source | PubChem | |
URL | https://pubchem.ncbi.nlm.nih.gov | |
Description | Data deposited in or computed by PubChem | |
DSSTOX Substance ID |
DTXSID80396837 | |
Record name | 4-Cyanoindole | |
Source | EPA DSSTox | |
URL | https://comptox.epa.gov/dashboard/DTXSID80396837 | |
Description | DSSTox provides a high quality public chemistry resource for supporting improved predictive toxicology. | |
Molecular Weight |
142.16 g/mol | |
Source | PubChem | |
URL | https://pubchem.ncbi.nlm.nih.gov | |
Description | Data deposited in or computed by PubChem | |
CAS No. |
16136-52-0 | |
Record name | 4-Cyanoindole | |
Source | EPA DSSTox | |
URL | https://comptox.epa.gov/dashboard/DTXSID80396837 | |
Description | DSSTox provides a high quality public chemistry resource for supporting improved predictive toxicology. | |
Record name | 4-Cyanoindole | |
Source | European Chemicals Agency (ECHA) | |
URL | https://echa.europa.eu/information-on-chemicals | |
Description | The European Chemicals Agency (ECHA) is an agency of the European Union which is the driving force among regulatory authorities in implementing the EU's groundbreaking chemicals legislation for the benefit of human health and the environment as well as for innovation and competitiveness. | |
Explanation | Use of the information, documents and data from the ECHA website is subject to the terms and conditions of this Legal Notice, and subject to other binding limitations provided for under applicable law, the information, documents and data made available on the ECHA website may be reproduced, distributed and/or used, totally or in part, for non-commercial purposes provided that ECHA is acknowledged as the source: "Source: European Chemicals Agency, http://echa.europa.eu/". Such acknowledgement must be included in each copy of the material. ECHA permits and encourages organisations and individuals to create links to the ECHA website under the following cumulative conditions: Links can only be made to webpages that provide a link to the Legal Notice page. | |
Retrosynthesis Analysis
AI-Powered Synthesis Planning: Our tool employs the Template_relevance Pistachio, Template_relevance Bkms_metabolic, Template_relevance Pistachio_ringbreaker, Template_relevance Reaxys, Template_relevance Reaxys_biocatalysis model, leveraging a vast database of chemical reactions to predict feasible synthetic routes.
One-Step Synthesis Focus: Specifically designed for one-step synthesis, it provides concise and direct routes for your target compounds, streamlining the synthesis process.
Accurate Predictions: Utilizing the extensive PISTACHIO, BKMS_METABOLIC, PISTACHIO_RINGBREAKER, REAXYS, REAXYS_BIOCATALYSIS database, our tool offers high-accuracy predictions, reflecting the latest in chemical research and data.
Strategy Settings
Precursor scoring | Relevance Heuristic |
---|---|
Min. plausibility | 0.01 |
Model | Template_relevance |
Template Set | Pistachio/Bkms_metabolic/Pistachio_ringbreaker/Reaxys/Reaxys_biocatalysis |
Top-N result to add to graph | 6 |
Feasible Synthetic Routes
試験管内研究製品の免責事項と情報
BenchChemで提示されるすべての記事および製品情報は、情報提供を目的としています。BenchChemで購入可能な製品は、生体外研究のために特別に設計されています。生体外研究は、ラテン語の "in glass" に由来し、生物体の外で行われる実験を指します。これらの製品は医薬品または薬として分類されておらず、FDAから任何の医療状態、病気、または疾患の予防、治療、または治癒のために承認されていません。これらの製品を人間または動物に体内に導入する形態は、法律により厳格に禁止されています。これらのガイドラインに従うことは、研究と実験において法的および倫理的な基準の遵守を確実にするために重要です。