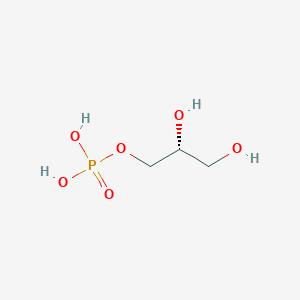
sn-グリセロール3-リン酸
概要
説明
sn-グリセロール3-リン酸: は、化学式HOCH₂CH(OH)CH₂OPO₃²⁻を持つ有機イオンです。これは、二塩基性リン酸(HOPO₃²⁻)とグリセロールのエステルの2つの立体異性体の1つです。 この化合物は、細菌および真核生物のグリセロリン脂質の重要な成分です 。これは、解糖系や脂質生合成など、さまざまな代謝経路で重要な役割を果たしています。
科学的研究の応用
Lipid Biosynthesis
sn-Glycerol 3-phosphate serves as a precursor for the synthesis of glycerolipids, which are essential components of cellular membranes. The enzyme glycerol-3-phosphate acyltransferase (GPAT) catalyzes the acylation of G3P to produce lysophosphatidic acid (LPA), a crucial intermediate in lipid metabolism.
Enzymatic Activity
- GPAT Enzymes : Different GPAT isoforms exist across various organisms, including plants and mammals. In plants, GPATs are involved in the biosynthesis of storage and membrane lipids, contributing to plant development and stress responses .
Organism | GPAT Isoform | Function |
---|---|---|
Arabidopsis thaliana | GPAT1, GPAT4, GPAT5 | Involved in cutin and suberin biosynthesis |
Escherichia coli | GlpT | G3P transport across the membrane |
Mammals | GPAT2 | Regulation of lipid metabolism and energy balance |
Physiological Roles
The physiological roles of G3P extend beyond lipid synthesis. It plays a part in signaling pathways that regulate cell growth and differentiation. For instance, LPA derived from G3P is known to activate various signaling cascades that influence cellular responses to environmental stimuli .
Metabolic Engineering
sn-Glycerol 3-phosphate is increasingly utilized in metabolic engineering to enhance lipid production for biofuels and pharmaceuticals. By manipulating pathways involving G3P, researchers aim to increase the yield of valuable lipids.
Case Studies
- Microbial Production : In engineered strains of Pichia pastoris, G3P is used as a substrate to produce high-value fatty acids like ricinoleic acid, demonstrating the potential for sustainable lipid production .
- Lipid Accumulation : Studies have shown that overexpression of GPAT genes can lead to increased lipid accumulation in microbial systems, which is vital for biofuel production .
Therapeutic Applications
G3P has therapeutic implications due to its role in cellular metabolism and signaling.
Diabetes Research
Research indicates that G3P levels correlate with insulin sensitivity. Elevated G3P may interfere with insulin signaling pathways, making it a target for diabetes treatment strategies .
Cancer Research
G3P is also being studied for its role in cancer metabolism. It has been observed that cancer cells exhibit altered G3P metabolism, which supports their rapid growth and survival under stress conditions .
Industrial Applications
Beyond biological functions, sn-Glycerol 3-phosphate has industrial applications.
Food Industry
G3P is used as a food additive and preservative due to its ability to retain moisture and improve texture in food products.
Cosmetic Industry
In cosmetics, G3P serves as a humectant, helping to maintain skin hydration and improve product stability.
作用機序
sn-グリセロール3-リン酸は、主に代謝経路における役割を通じてその効果を発揮します。これは、NADHからミトコンドリアの電子伝達系に電子を転移し、ATP産生を促進するグリセロリン酸シャトルに関与しています。 これは、細胞膜の完全性と機能に不可欠なグリセロ脂質の合成の前駆体としても役立ちます .
生化学分析
Biochemical Properties
sn-Glycerol 3-phosphate plays a pivotal role in biochemical reactions. It is synthesized by reducing dihydroxyacetone phosphate (DHAP), an intermediate in glycolysis, through the action of glycerol-3-phosphate dehydrogenase . It can also be synthesized from amino acids and citric acid cycle intermediates via the glyceroneogenesis pathway . sn-Glycerol 3-phosphate interacts with various enzymes, proteins, and other biomolecules. For instance, it is first acylated on its sn-1 position by an ER- or mitochondrial membrane enzyme, glycerol-3-phosphate O-acyltransferase .
Cellular Effects
sn-Glycerol 3-phosphate has significant effects on various types of cells and cellular processes. It influences cell function by acting as a major link between carbohydrate metabolism and lipid metabolism . It is also a major contributor of electrons to the electron transport chain in the mitochondria .
Molecular Mechanism
The molecular mechanism of sn-Glycerol 3-phosphate involves its conversion to dihydroxyacetone phosphate (DHAP) by glycerol-3-phosphate dehydrogenase . This enzyme is located both in the cytosol and the intermembrane face of the mitochondrial inner membrane . The conversion process involves the reduction of FAD to FADH2, consequently transferring electrons to coenzyme Q (CoQ) in the electron transport chain .
Temporal Effects in Laboratory Settings
It is known that sn-Glycerol 3-phosphate is a starting material for de novo synthesis of glycerolipids , suggesting that its effects on cellular function may be long-term and dependent on the stability and degradation of these lipids.
Dosage Effects in Animal Models
It is known that sn-Glycerol 3-phosphate plays a critical role in the development of obesity, hepatic steatosis, and insulin resistance , suggesting that its effects may vary with dosage.
Metabolic Pathways
sn-Glycerol 3-phosphate is involved in several metabolic pathways. It is a starting material for de novo synthesis of glycerolipids . It can also enter the glycerol phosphate shuttle to generate NAD+, or be converted to glyceraldehyde 3-phosphate and enter glycolysis or the lipid biosynthesis pathway .
Transport and Distribution
sn-Glycerol 3-phosphate and dihydroxyacetone phosphate (DHAP) are molecules so small that they can permeate the mitochondrial outer membrane through porins and shuttle between two dehydrogenases . This suggests that sn-Glycerol 3-phosphate can be transported and distributed within cells and tissues.
Subcellular Localization
sn-Glycerol 3-phosphate dehydrogenases, the enzymes that interact with sn-Glycerol 3-phosphate, are located both in the cytosol and the intermembrane face of the mitochondrial inner membrane . This suggests that sn-Glycerol 3-phosphate may also be localized in these areas within the cell.
準備方法
合成経路と反応条件:
ジヒドロキシアセトンリン酸(DHAP)の還元: この方法は、解糖系の中間体であるDHAPの還元を、グリセロール-3-リン酸デヒドロゲナーゼによって触媒する方法です。反応は以下のとおりです。 [ \text{DHAP} + \text{NAD(P)H} + \text{H}^+ \rightarrow \text{sn-グリセロール3-リン酸} + \text{NAD(P)}^+ ]
グリセロールのリン酸化: グリセロールは、グリセロールキナーゼによってリン酸化され、sn-グリセロール3-リン酸を生成します。この反応は以下のとおりです。 [ \text{グリセロール} + \text{ATP} \rightarrow \text{sn-グリセロール3-リン酸} + \text{ADP} ]
工業的生産方法: 工業的生産では、その特異性と効率性から、酵素的方法がしばしば用いられます。 バイオリアクターにおけるグリセロール-3-リン酸デヒドロゲナーゼとグリセロールキナーゼの使用は一般的です .
化学反応の分析
反応の種類:
酸化: sn-グリセロール3-リン酸は、グリセロール-3-リン酸デヒドロゲナーゼによってジヒドロキシアセトンリン酸(DHAP)に酸化されます。
アシル化: これは、リゾホスファチジン酸を形成するためにアシル化され、さらにアシル化されてホスファチジン酸を生成します。
一般的な試薬と条件:
酸化: グリセロール-3-リン酸デヒドロゲナーゼ、NAD⁺またはNADP⁺。
アシル化: アシル-CoAとグリセロール-3-リン酸O-アシル転移酵素。
主な生成物:
酸化: ジヒドロキシアセトンリン酸(DHAP)。
アシル化: リゾホスファチジン酸とホスファチジン酸.
科学研究への応用
化学:
- グリセロ脂質の合成の出発物質として使用されます。
生物学:
医学:
産業:
類似化合物との比較
類似化合物:
グリセロール1-リン酸: グリセロールリン酸の別の立体異性体。
グリセロール2-リン酸: あまり一般的ではない異性体。
ユニークさ: sn-グリセロール3-リン酸は、グリセロリン酸シャトルにおける特異的な役割とグリセロ脂質の合成への関与により、ユニークです。 その立体化学により、その異性体では不可能な特定の酵素反応に参加することができます .
生物活性
sn-Glycerol 3-phosphate (G3P) is a crucial metabolite involved in various biological processes, including lipid metabolism, energy production, and signaling pathways. This article explores the biological activity of G3P, emphasizing its enzymatic roles, metabolic pathways, and implications in health and disease.
Overview of sn-Glycerol 3-Phosphate
sn-Glycerol 3-phosphate is a glycerol phosphate that serves as an essential intermediate in lipid biosynthesis and energy metabolism. It is produced from glycerol through the action of glycerol kinase or from dihydroxyacetone phosphate (DHAP) via the action of glycerol-3-phosphate dehydrogenase (GPDH). G3P plays a pivotal role in the synthesis of phospholipids and triglycerides, which are vital components of cellular membranes and energy storage.
Enzymatic Functions
-
Glycerol-3-Phosphate Dehydrogenases :
- Mitochondrial GPDH (mGPDH) : This enzyme catalyzes the oxidation of G3P to DHAP, linking lipid metabolism with the mitochondrial electron transport chain. It is crucial for maintaining cellular energy homeostasis and regulating reactive oxygen species (ROS) production .
- Cytosolic GPDH : This enzyme functions predominantly in the cytosol, converting DHAP back to G3P, thus playing a role in gluconeogenesis and lipid synthesis .
- sn-Glycerol 3-Phosphate Acyltransferases (GPAT) :
Metabolic Pathways
sn-Glycerol 3-phosphate is integral to several metabolic pathways:
- Lipid Biosynthesis :
- Energy Production :
Case Study 1: Role in Cancer Metabolism
Recent studies have highlighted the role of G3P in cancer cell metabolism. Enhanced activity of mitochondrial GPDH has been associated with increased lipid oxidation and altered metabolic profiles in renal clear cell carcinoma cells. This suggests that targeting GPDH may provide therapeutic avenues for cancer treatment .
Case Study 2: Obesity and Insulin Resistance
Research indicates that elevated levels of cytosolic GPDH activity are linked to obesity and insulin resistance. In adipose tissues from obese individuals, increased glycerol-3-phosphate levels correlate with enhanced lipogenesis, contributing to fat accumulation .
Data Tables
Enzyme | Function | Location |
---|---|---|
Mitochondrial GPDH | Oxidation of G3P to DHAP | Mitochondria |
Cytosolic GPDH | Reduction of DHAP to G3P | Cytosol |
sn-Glycerol 3-Phosphate Acyltransferase (GPAT) | Acylation of G3P to produce LPA | Endoplasmic reticulum & mitochondria |
特性
IUPAC Name |
[(2R)-2,3-dihydroxypropyl] dihydrogen phosphate | |
---|---|---|
Source | PubChem | |
URL | https://pubchem.ncbi.nlm.nih.gov | |
Description | Data deposited in or computed by PubChem | |
InChI |
InChI=1S/C3H9O6P/c4-1-3(5)2-9-10(6,7)8/h3-5H,1-2H2,(H2,6,7,8)/t3-/m1/s1 | |
Source | PubChem | |
URL | https://pubchem.ncbi.nlm.nih.gov | |
Description | Data deposited in or computed by PubChem | |
InChI Key |
AWUCVROLDVIAJX-GSVOUGTGSA-N | |
Source | PubChem | |
URL | https://pubchem.ncbi.nlm.nih.gov | |
Description | Data deposited in or computed by PubChem | |
Canonical SMILES |
C(C(COP(=O)(O)O)O)O | |
Source | PubChem | |
URL | https://pubchem.ncbi.nlm.nih.gov | |
Description | Data deposited in or computed by PubChem | |
Isomeric SMILES |
C([C@H](COP(=O)(O)O)O)O | |
Source | PubChem | |
URL | https://pubchem.ncbi.nlm.nih.gov | |
Description | Data deposited in or computed by PubChem | |
Molecular Formula |
C3H9O6P | |
Source | PubChem | |
URL | https://pubchem.ncbi.nlm.nih.gov | |
Description | Data deposited in or computed by PubChem | |
DSSTOX Substance ID |
DTXSID5048346 | |
Record name | sn-Glycerol 3-phosphate | |
Source | EPA DSSTox | |
URL | https://comptox.epa.gov/dashboard/DTXSID5048346 | |
Description | DSSTox provides a high quality public chemistry resource for supporting improved predictive toxicology. | |
Molecular Weight |
172.07 g/mol | |
Source | PubChem | |
URL | https://pubchem.ncbi.nlm.nih.gov | |
Description | Data deposited in or computed by PubChem | |
Physical Description |
Solid | |
Record name | Glycerol 3-phosphate | |
Source | Human Metabolome Database (HMDB) | |
URL | http://www.hmdb.ca/metabolites/HMDB0000126 | |
Description | The Human Metabolome Database (HMDB) is a freely available electronic database containing detailed information about small molecule metabolites found in the human body. | |
Explanation | HMDB is offered to the public as a freely available resource. Use and re-distribution of the data, in whole or in part, for commercial purposes requires explicit permission of the authors and explicit acknowledgment of the source material (HMDB) and the original publication (see the HMDB citing page). We ask that users who download significant portions of the database cite the HMDB paper in any resulting publications. | |
Solubility |
1000.0 mg/mL | |
Record name | Glycerol 3-phosphate | |
Source | Human Metabolome Database (HMDB) | |
URL | http://www.hmdb.ca/metabolites/HMDB0000126 | |
Description | The Human Metabolome Database (HMDB) is a freely available electronic database containing detailed information about small molecule metabolites found in the human body. | |
Explanation | HMDB is offered to the public as a freely available resource. Use and re-distribution of the data, in whole or in part, for commercial purposes requires explicit permission of the authors and explicit acknowledgment of the source material (HMDB) and the original publication (see the HMDB citing page). We ask that users who download significant portions of the database cite the HMDB paper in any resulting publications. | |
CAS No. |
17989-41-2, 57-03-4 | |
Record name | Glycerol 3-phosphate | |
Source | CAS Common Chemistry | |
URL | https://commonchemistry.cas.org/detail?cas_rn=17989-41-2 | |
Description | CAS Common Chemistry is an open community resource for accessing chemical information. Nearly 500,000 chemical substances from CAS REGISTRY cover areas of community interest, including common and frequently regulated chemicals, and those relevant to high school and undergraduate chemistry classes. This chemical information, curated by our expert scientists, is provided in alignment with our mission as a division of the American Chemical Society. | |
Explanation | The data from CAS Common Chemistry is provided under a CC-BY-NC 4.0 license, unless otherwise stated. | |
Record name | Glyceryl 1-phosphate, (R)- | |
Source | ChemIDplus | |
URL | https://pubchem.ncbi.nlm.nih.gov/substance/?source=chemidplus&sourceid=0017989412 | |
Description | ChemIDplus is a free, web search system that provides access to the structure and nomenclature authority files used for the identification of chemical substances cited in National Library of Medicine (NLM) databases, including the TOXNET system. | |
Record name | sn-glycerol 3-phosphate | |
Source | DrugBank | |
URL | https://www.drugbank.ca/drugs/DB02515 | |
Description | The DrugBank database is a unique bioinformatics and cheminformatics resource that combines detailed drug (i.e. chemical, pharmacological and pharmaceutical) data with comprehensive drug target (i.e. sequence, structure, and pathway) information. | |
Explanation | Creative Common's Attribution-NonCommercial 4.0 International License (http://creativecommons.org/licenses/by-nc/4.0/legalcode) | |
Record name | sn-Glycerol 3-phosphate | |
Source | EPA DSSTox | |
URL | https://comptox.epa.gov/dashboard/DTXSID5048346 | |
Description | DSSTox provides a high quality public chemistry resource for supporting improved predictive toxicology. | |
Record name | GLYCERYL 1-PHOSPHATE, (R)- | |
Source | FDA Global Substance Registration System (GSRS) | |
URL | https://gsrs.ncats.nih.gov/ginas/app/beta/substances/370V52HE4B | |
Description | The FDA Global Substance Registration System (GSRS) enables the efficient and accurate exchange of information on what substances are in regulated products. Instead of relying on names, which vary across regulatory domains, countries, and regions, the GSRS knowledge base makes it possible for substances to be defined by standardized, scientific descriptions. | |
Explanation | Unless otherwise noted, the contents of the FDA website (www.fda.gov), both text and graphics, are not copyrighted. They are in the public domain and may be republished, reprinted and otherwise used freely by anyone without the need to obtain permission from FDA. Credit to the U.S. Food and Drug Administration as the source is appreciated but not required. | |
Record name | Glycerol 3-phosphate | |
Source | Human Metabolome Database (HMDB) | |
URL | http://www.hmdb.ca/metabolites/HMDB0000126 | |
Description | The Human Metabolome Database (HMDB) is a freely available electronic database containing detailed information about small molecule metabolites found in the human body. | |
Explanation | HMDB is offered to the public as a freely available resource. Use and re-distribution of the data, in whole or in part, for commercial purposes requires explicit permission of the authors and explicit acknowledgment of the source material (HMDB) and the original publication (see the HMDB citing page). We ask that users who download significant portions of the database cite the HMDB paper in any resulting publications. | |
Melting Point |
102 - 104 °C | |
Record name | Glycerol 3-phosphate | |
Source | Human Metabolome Database (HMDB) | |
URL | http://www.hmdb.ca/metabolites/HMDB0000126 | |
Description | The Human Metabolome Database (HMDB) is a freely available electronic database containing detailed information about small molecule metabolites found in the human body. | |
Explanation | HMDB is offered to the public as a freely available resource. Use and re-distribution of the data, in whole or in part, for commercial purposes requires explicit permission of the authors and explicit acknowledgment of the source material (HMDB) and the original publication (see the HMDB citing page). We ask that users who download significant portions of the database cite the HMDB paper in any resulting publications. | |
Synthesis routes and methods
Procedure details
Retrosynthesis Analysis
AI-Powered Synthesis Planning: Our tool employs the Template_relevance Pistachio, Template_relevance Bkms_metabolic, Template_relevance Pistachio_ringbreaker, Template_relevance Reaxys, Template_relevance Reaxys_biocatalysis model, leveraging a vast database of chemical reactions to predict feasible synthetic routes.
One-Step Synthesis Focus: Specifically designed for one-step synthesis, it provides concise and direct routes for your target compounds, streamlining the synthesis process.
Accurate Predictions: Utilizing the extensive PISTACHIO, BKMS_METABOLIC, PISTACHIO_RINGBREAKER, REAXYS, REAXYS_BIOCATALYSIS database, our tool offers high-accuracy predictions, reflecting the latest in chemical research and data.
Strategy Settings
Precursor scoring | Relevance Heuristic |
---|---|
Min. plausibility | 0.01 |
Model | Template_relevance |
Template Set | Pistachio/Bkms_metabolic/Pistachio_ringbreaker/Reaxys/Reaxys_biocatalysis |
Top-N result to add to graph | 6 |
Feasible Synthetic Routes
Q1: What is sn-glycerol 3-phosphate and why is it important?
A: sn-Glycerol 3-phosphate (sn-glycerol-3P) is a key intermediate in several metabolic pathways, playing a critical role in both lipid and carbohydrate metabolism. It serves as a precursor for the biosynthesis of phospholipids, the building blocks of cell membranes, and triacylglycerols, a major form of energy storage. [, , , ] Additionally, it participates in the transport of reducing equivalents between cellular compartments. [, ]
Q2: How does sn-glycerol 3-phosphate contribute to phospholipid biosynthesis?
A: sn-Glycerol 3-phosphate is acylated by acyl-CoA-sn-glycerol 3-phosphate O-acyltransferase (EC 2.3.1.15), also known as glycerol-3-phosphate acyltransferase, in a two-step process. The first step involves the acylation of sn-glycerol 3-phosphate at the sn-1 position, primarily with saturated fatty acids like palmitate and stearate, to form lysophosphatidic acid (1-acyl-sn-glycerol 3-phosphate). The second step involves the acylation of lysophosphatidic acid at the sn-2 position, generally with unsaturated fatty acids like linoleate, to form phosphatidic acid (1,2-diacyl-sn-glycerol 3-phosphate). Phosphatidic acid is a key precursor for the synthesis of various phospholipids, including phosphatidylcholine and phosphatidylethanolamine, through the Kennedy pathway. [, , , , ]
Q3: What determines the fatty acid composition of phosphatidic acid?
A: The fatty acid composition of phosphatidic acid, and subsequently other phospholipids, is determined by the substrate specificity of the acyltransferases involved in its synthesis. [, , ] Different isoforms of glycerol-3-phosphate acyltransferase exhibit distinct fatty acid preferences, contributing to the diversity of phospholipid structures found in various tissues and organisms. For instance, in the developing cotyledons of safflower (Carthamus tinctorius L.) seeds, the sn-1 position of phosphatidic acid is predominantly esterified with saturated fatty acids, while the sn-2 position favors unsaturated fatty acids like linoleate. [] This non-random distribution of acyl groups is crucial for regulating the fluidity and function of cellular membranes.
Q4: Besides phospholipids, what other important lipids are synthesized from sn-glycerol 3-phosphate?
A: sn-Glycerol 3-phosphate is a crucial precursor for triacylglycerol synthesis, a major energy reserve in many organisms. [, , ] Following the formation of phosphatidic acid, a specific phosphatase, phosphatidate phosphohydrolase, removes the phosphate group from phosphatidic acid, generating diacylglycerol. [, ] Diacylglycerol is then acylated by diacylglycerol acyltransferase, utilizing acyl-CoA as the acyl donor, to form triacylglycerol. []
Q5: How does sn-glycerol 3-phosphate contribute to energy metabolism?
A: sn-Glycerol 3-phosphate participates in the transport of reducing equivalents, specifically NADH, generated during glycolysis in the cytosol, across the mitochondrial membrane for oxidative phosphorylation and ATP production. [, , ] This shuttle system, known as the glycerol-3-phosphate shuttle, involves the interconversion of dihydroxyacetone phosphate and sn-glycerol 3-phosphate by two isoforms of glycerol-3-phosphate dehydrogenase: a cytosolic NAD+-dependent isoform and a mitochondrial FAD-linked isoform.
Q6: How is the sn-glycerol 3-phosphate level regulated in cells?
A: The intracellular concentration of sn-glycerol 3-phosphate is tightly regulated by the balance between its synthesis and utilization in various metabolic pathways. [, ] Enzymes like glycerol-3-phosphate dehydrogenase and glycerol-3-phosphate acyltransferase are subject to allosteric regulation by metabolites, such as sn-glycerol 3-phosphate itself, fatty acyl-CoAs, and ATP, as well as hormonal control, which fine-tune sn-glycerol 3-phosphate levels to meet cellular demands. [, , , ]
Q7: How does ethanol consumption affect sn-glycerol 3-phosphate metabolism in the liver?
A: Ethanol metabolism in the liver leads to a significant increase in the NADH/NAD+ ratio, which in turn promotes the reduction of dihydroxyacetone phosphate to sn-glycerol 3-phosphate, resulting in its accumulation. [, , , ] This increase in hepatic sn-glycerol 3-phosphate levels can contribute to several metabolic disturbances associated with alcohol consumption, including fatty liver disease.
Q8: Are there any specific inhibitors of sn-glycerol 3-phosphate metabolism?
A: Phenethyl alcohol has been shown to inhibit sn-glycerol 3-phosphate acyltransferase, the enzyme responsible for the first step in phospholipid synthesis. [] This inhibition leads to a decrease in phospholipid production, highlighting the importance of sn-glycerol 3-phosphate acylation for cell membrane biogenesis.
Q9: What is the role of sn-glycerol 3-phosphate in microorganisms like E. coli?
A: Similar to mammalian cells, sn-glycerol 3-phosphate is essential for phospholipid biosynthesis in bacteria like E. coli. [, , , , ] E. coli possesses a specific transport system for sn-glycerol 3-phosphate uptake, highlighting its importance as a precursor molecule. [, ] Inhibition of sn-glycerol 3-phosphate metabolism in E. coli can have significant consequences for cell growth and survival. [, ]
Q10: Are there any known diseases associated with defects in sn-glycerol 3-phosphate metabolism?
A: While specific diseases directly linked to sn-glycerol 3-phosphate metabolism are rare, genetic variations in genes encoding enzymes involved in its metabolism, such as AGPAT2, have been associated with altered lipid metabolism and disease susceptibility in humans. [] Further research is needed to fully elucidate the link between sn-glycerol 3-phosphate metabolism and human diseases.
試験管内研究製品の免責事項と情報
BenchChemで提示されるすべての記事および製品情報は、情報提供を目的としています。BenchChemで購入可能な製品は、生体外研究のために特別に設計されています。生体外研究は、ラテン語の "in glass" に由来し、生物体の外で行われる実験を指します。これらの製品は医薬品または薬として分類されておらず、FDAから任何の医療状態、病気、または疾患の予防、治療、または治癒のために承認されていません。これらの製品を人間または動物に体内に導入する形態は、法律により厳格に禁止されています。これらのガイドラインに従うことは、研究と実験において法的および倫理的な基準の遵守を確実にするために重要です。