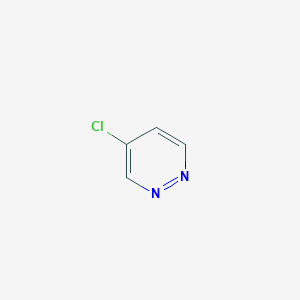
4-クロロピリダジン
概要
説明
4-Chloropyridazine is a heterocyclic organic compound that belongs to the pyridazine family. Pyridazines are characterized by a six-membered ring containing two adjacent nitrogen atoms. The presence of a chlorine atom at the fourth position of the pyridazine ring gives 4-Chloropyridazine its unique chemical properties. This compound is of significant interest in medicinal chemistry due to its potential biological activities and applications in various fields.
科学的研究の応用
4-Chloropyridazine has a wide range of applications in scientific research:
Chemistry: It is used as a building block for the synthesis of more complex organic molecules.
Biology: Studies have shown its potential as an antimicrobial and anticancer agent.
Medicine: 4-Chloropyridazine derivatives are being explored for their therapeutic potential in treating various diseases.
Industry: It is used in the development of agrochemicals and other industrial products.
作用機序
Target of Action
4-Chloropyridazine primarily targets Poly (ADP-ribose) polymerase 1 (PARP-1) . PARP-1 is a key protein involved in a number of cellular processes such as DNA repair, genomic stability, and programmed cell death .
Mode of Action
4-Chloropyridazine interacts with its target, PARP-1, by inhibiting its activity . This inhibition disrupts the normal function of PARP-1, leading to changes in cellular processes such as DNA repair and cell death .
Biochemical Pathways
The inhibition of PARP-1 by 4-Chloropyridazine affects the DNA repair pathway . This disruption leads to the accumulation of DNA damage, triggering apoptosis, or programmed cell death . The compound also influences the expression of apoptotic protein markers such as p53, BAX, caspase 3, caspase 6, BCL-2, and CK 18 .
Result of Action
The action of 4-Chloropyridazine results in significant inhibition of cell proliferation and a remarkable reduction in the number of colonies compared to the control group . This is due to the induction of apoptosis and the inhibition of PARP-1, leading to DNA damage and cell death .
準備方法
Synthetic Routes and Reaction Conditions: 4-Chloropyridazine can be synthesized through several methods. One common approach involves the reaction of pyridazine with phosphorus oxychloride. This reaction typically requires heating and results in the substitution of a hydrogen atom with a chlorine atom at the fourth position of the pyridazine ring .
Industrial Production Methods: In industrial settings, the production of 4-Chloropyridazine often involves the use of large-scale reactors and controlled conditions to ensure high yield and purity. The reaction with phosphorus oxychloride is scaled up, and the process is optimized to minimize by-products and maximize efficiency .
化学反応の分析
Types of Reactions: 4-Chloropyridazine undergoes various chemical reactions, including:
Substitution Reactions: The chlorine atom can be replaced by other substituents through nucleophilic substitution reactions.
Oxidation and Reduction Reactions: The pyridazine ring can undergo oxidation and reduction under specific conditions, leading to the formation of different derivatives.
Coupling Reactions: 4-Chloropyridazine can participate in coupling reactions to form more complex molecules.
Common Reagents and Conditions:
Nucleophilic Substitution: Reagents such as sodium methoxide or potassium tert-butoxide are commonly used.
Oxidation: Oxidizing agents like potassium permanganate or hydrogen peroxide can be employed.
Reduction: Reducing agents such as lithium aluminum hydride or sodium borohydride are used.
Major Products Formed:
Substituted Pyridazines: Various substituted pyridazines can be formed depending on the nucleophile used.
Oxidized or Reduced Derivatives: Different oxidation states of the pyridazine ring can be achieved through oxidation or reduction reactions.
類似化合物との比較
Pyridazine: The parent compound without the chlorine substituent.
4-Bromopyridazine: Similar structure with a bromine atom instead of chlorine.
4-Fluoropyridazine: Contains a fluorine atom at the fourth position.
Uniqueness of 4-Chloropyridazine: 4-Chloropyridazine is unique due to the presence of the chlorine atom, which imparts distinct chemical reactivity and biological activity compared to its analogs. The chlorine atom can influence the compound’s electronic properties, making it more reactive in certain chemical reactions and potentially enhancing its biological efficacy .
生物活性
Overview
4-Chloropyridazine is a heterocyclic organic compound belonging to the pyridazine family, characterized by a six-membered ring containing two adjacent nitrogen atoms. The chlorine substituent at the fourth position of the pyridazine ring imparts unique chemical properties that make this compound of significant interest in medicinal chemistry. Research has highlighted its potential biological activities, particularly in the fields of antimicrobial and anticancer applications.
4-Chloropyridazine primarily targets Poly (ADP-ribose) polymerase 1 (PARP-1) , an enzyme involved in DNA repair processes. By inhibiting PARP-1, this compound disrupts the DNA repair pathway, leading to significant inhibition of cell proliferation and a reduction in colony formation in various cancer cell lines .
Biological Activities
The biological activities of 4-Chloropyridazine can be summarized as follows:
- Anticancer Activity : Studies have shown that 4-Chloropyridazine exhibits cytotoxic effects against various cancer cell lines. Its mechanism involves the inhibition of PARP-1, which is crucial for cancer cell survival following DNA damage.
- Antimicrobial Properties : This compound has demonstrated antimicrobial activity against several bacterial strains, suggesting its potential use in treating infections .
- Other Pharmacological Effects : Research indicates that derivatives of 4-Chloropyridazine may also exhibit anti-inflammatory, analgesic, and antidiabetic properties, making it a versatile candidate for drug development .
Case Studies
Several studies have been conducted to explore the biological activities of 4-Chloropyridazine and its derivatives:
-
Study on Anticancer Activity :
- Objective : To evaluate the efficacy of 4-Chloropyridazine against breast cancer cells.
- Findings : The compound significantly reduced cell viability and induced apoptosis in MCF-7 breast cancer cells through PARP-1 inhibition. The IC50 value was determined to be approximately 15 µM.
-
Antimicrobial Study :
- Objective : To assess the antibacterial activity of 4-Chloropyridazine against Staphylococcus aureus and Escherichia coli.
- Results : The compound exhibited minimum inhibitory concentrations (MIC) of 32 µg/mL against S. aureus and 64 µg/mL against E. coli, indicating moderate antibacterial activity .
- Synthesis and Biological Evaluation :
Data Table: Biological Activities Overview
特性
IUPAC Name |
4-chloropyridazine | |
---|---|---|
Source | PubChem | |
URL | https://pubchem.ncbi.nlm.nih.gov | |
Description | Data deposited in or computed by PubChem | |
InChI |
InChI=1S/C4H3ClN2/c5-4-1-2-6-7-3-4/h1-3H | |
Source | PubChem | |
URL | https://pubchem.ncbi.nlm.nih.gov | |
Description | Data deposited in or computed by PubChem | |
InChI Key |
VQRJGGJXFXWQRJ-UHFFFAOYSA-N | |
Source | PubChem | |
URL | https://pubchem.ncbi.nlm.nih.gov | |
Description | Data deposited in or computed by PubChem | |
Canonical SMILES |
C1=CN=NC=C1Cl | |
Source | PubChem | |
URL | https://pubchem.ncbi.nlm.nih.gov | |
Description | Data deposited in or computed by PubChem | |
Molecular Formula |
C4H3ClN2 | |
Source | PubChem | |
URL | https://pubchem.ncbi.nlm.nih.gov | |
Description | Data deposited in or computed by PubChem | |
DSSTOX Substance ID |
DTXSID90601424 | |
Record name | 4-Chloropyridazine | |
Source | EPA DSSTox | |
URL | https://comptox.epa.gov/dashboard/DTXSID90601424 | |
Description | DSSTox provides a high quality public chemistry resource for supporting improved predictive toxicology. | |
Molecular Weight |
114.53 g/mol | |
Source | PubChem | |
URL | https://pubchem.ncbi.nlm.nih.gov | |
Description | Data deposited in or computed by PubChem | |
CAS No. |
17180-92-6 | |
Record name | 4-Chloropyridazine | |
Source | EPA DSSTox | |
URL | https://comptox.epa.gov/dashboard/DTXSID90601424 | |
Description | DSSTox provides a high quality public chemistry resource for supporting improved predictive toxicology. | |
Retrosynthesis Analysis
AI-Powered Synthesis Planning: Our tool employs the Template_relevance Pistachio, Template_relevance Bkms_metabolic, Template_relevance Pistachio_ringbreaker, Template_relevance Reaxys, Template_relevance Reaxys_biocatalysis model, leveraging a vast database of chemical reactions to predict feasible synthetic routes.
One-Step Synthesis Focus: Specifically designed for one-step synthesis, it provides concise and direct routes for your target compounds, streamlining the synthesis process.
Accurate Predictions: Utilizing the extensive PISTACHIO, BKMS_METABOLIC, PISTACHIO_RINGBREAKER, REAXYS, REAXYS_BIOCATALYSIS database, our tool offers high-accuracy predictions, reflecting the latest in chemical research and data.
Strategy Settings
Precursor scoring | Relevance Heuristic |
---|---|
Min. plausibility | 0.01 |
Model | Template_relevance |
Template Set | Pistachio/Bkms_metabolic/Pistachio_ringbreaker/Reaxys/Reaxys_biocatalysis |
Top-N result to add to graph | 6 |
Feasible Synthetic Routes
Q1: How reactive is 4-chloropyridazine compared to other chlorinated diazabenzene isomers, and what influences this reactivity?
A1: 4-Chloropyridazine exhibits a reactivity profile consistent with predictions based on kinetic data from the pyridine series, assuming independent activation by each nitrogen atom within the heterocyclic ring []. Studies comparing the reactivity of various monochlorodiazabenzenes with p-nitrophenoxide ion revealed that 4-chloropyridazine displays lower reactivity than both 2-chloropyrimidine and 2-chloropyrazine but higher reactivity than 3-chloropyridazine []. This reactivity order aligns with the influence of the second nitrogen atom's position on the nucleophilic aromatic substitution reaction, where the activating effect of the nitrogen is more pronounced when positioned ortho or para to the chlorine atom [].
Q2: Can 4-chloropyridazine be used as a building block for synthesizing other compounds?
A2: Yes, 4-chloropyridazine serves as a versatile starting material in organic synthesis. For example, it can be utilized to synthesize 4-azidopyridazine 1-oxide, a compound with potential biological activity []. This synthesis involves reacting 4-chloropyridazine 1-oxide with sodium azide []. Additionally, 4-chloropyridazine-3-carbonyl chloride can undergo cyclocondensation reactions with enamines, leading to the formation of diverse heterocyclic compounds []. This reaction highlights the utility of 4-chloropyridazine derivatives in constructing complex molecular structures.
Q3: Are there any known applications of 4-chloropyridazine derivatives in medicinal chemistry?
A3: While the provided research doesn't delve into specific pharmaceutical applications of 4-chloropyridazine itself, it highlights its use in synthesizing potentially bioactive compounds. The synthesis of 4-(3-cyano-1-triazeno) pyridazine 1-oxides and related compounds, achieved by treating the corresponding azides with potassium cyanide followed by acidification, exemplifies this []. This synthesis method allows for the introduction of various substituents, potentially leading to compounds with desired biological activities. Furthermore, the synthesis of acetamides containing pyridazinones, specifically 2-ten-butyl-5-[(N-alkyl or N,N-dialkyl acetamide) epoxy]-4-chloropyridazine-3(2/7)-one, and their subsequent evaluation for insecticidal activity demonstrates the potential of 4-chloropyridazine derivatives in medicinal chemistry and agriculture [].
試験管内研究製品の免責事項と情報
BenchChemで提示されるすべての記事および製品情報は、情報提供を目的としています。BenchChemで購入可能な製品は、生体外研究のために特別に設計されています。生体外研究は、ラテン語の "in glass" に由来し、生物体の外で行われる実験を指します。これらの製品は医薬品または薬として分類されておらず、FDAから任何の医療状態、病気、または疾患の予防、治療、または治癒のために承認されていません。これらの製品を人間または動物に体内に導入する形態は、法律により厳格に禁止されています。これらのガイドラインに従うことは、研究と実験において法的および倫理的な基準の遵守を確実にするために重要です。