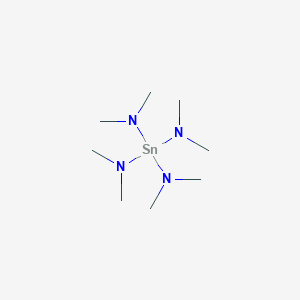
テトラキス(ジメチルアミノ)スズ
概要
説明
Tetrakis(dimethylamino)tin is an organotin compound with the chemical formula [(CH₃)₂N]₄Sn. It is a colorless liquid that is used primarily in the field of materials science and organometallic chemistry. The compound is known for its volatility and reactivity, making it a valuable precursor in various chemical processes.
科学的研究の応用
Tetrakis(dimethylamino)tin has several applications in scientific research:
Materials Science: Used as a precursor for the deposition of tin-containing thin films and coatings.
Organometallic Chemistry: Serves as a reagent in the synthesis of various organotin compounds.
Catalysis: Acts as a catalyst or catalyst precursor in organic reactions.
Semiconductor Industry: Utilized in the production of tin-based semiconductors and electronic materials.
作用機序
Target of Action
Tetrakis(dimethylamino)tin, also known as Tetrakis(dimethylamido)tin(IV), is a metal-organic compound . Its primary targets are surfaces where it is used to deposit thin films in various industrial applications . The compound interacts with these surfaces to create a desired effect, such as the fabrication of transparent conducting tin oxide (SnO2) thin films .
Mode of Action
The compound’s mode of action is primarily through a process known as Atomic Layer Deposition (ALD) . In this process, Tetrakis(dimethylamino)tin serves as a precursor, reacting with the surface of the target material to deposit a thin film . The exact nature of this interaction depends on the specific conditions of the ALD process, such as temperature and pressure .
Biochemical Pathways
While Tetrakis(dimethylamino)tin is not typically involved in biochemical pathways due to its industrial use, its chemical reactions during the ALD process can be considered analogous to a pathway. The compound reacts with the surface of the target material, leading to the deposition of a thin film. This reaction is the primary “pathway” through which Tetrakis(dimethylamino)tin exerts its effects .
Pharmacokinetics
Its properties in terms of reactivity, volatility, and stability are crucial for its function in ald processes .
Result of Action
The primary result of Tetrakis(dimethylamino)tin’s action is the deposition of thin films on target surfaces . These films can have various properties depending on the specifics of the ALD process and the nature of the target surface . For example, the compound has been used to fabricate transparent conducting tin oxide (SnO2) thin films .
Action Environment
The action of Tetrakis(dimethylamino)tin is heavily influenced by environmental factors. The ALD process, in which the compound is used, is sensitive to conditions such as temperature and pressure . Additionally, the compound is sensitive to moisture, requiring careful handling and storage .
準備方法
Synthetic Routes and Reaction Conditions
Tetrakis(dimethylamino)tin can be synthesized through the reaction of tin(IV) chloride with lithium dimethylamide. The reaction is typically carried out in an inert atmosphere to prevent the compound from reacting with moisture or oxygen. The general reaction is as follows:
SnCl4+4LiN(CH3)2→Sn[N(CH3)2]4+4LiCl
The reaction is exothermic and requires careful control of temperature and reaction conditions to ensure the purity of the product.
Industrial Production Methods
In industrial settings, the production of Tetrakis(dimethylamino)tin follows similar synthetic routes but on a larger scale. The process involves the use of high-purity reagents and advanced purification techniques to obtain the compound in its purest form. The compound is typically stored in airtight containers to prevent degradation.
化学反応の分析
Types of Reactions
Tetrakis(dimethylamino)tin undergoes various types of chemical reactions, including:
Oxidation: The compound can be oxidized to form tin oxides.
Hydrolysis: Reacts with water to form tin hydroxides and dimethylamine.
Substitution: Can undergo ligand exchange reactions with other amines or phosphines.
Common Reagents and Conditions
Oxidation: Common oxidizing agents include oxygen and hydrogen peroxide.
Hydrolysis: Water or aqueous solutions.
Substitution: Amines or phosphines under inert atmosphere conditions.
Major Products
Oxidation: Tin oxides.
Hydrolysis: Tin hydroxides and dimethylamine.
Substitution: New organotin compounds with different ligands.
類似化合物との比較
Similar Compounds
Tetrakis(diethylamido)tin(IV): Similar in structure but with ethyl groups instead of methyl groups.
Tetrakis(dimethylamido)titanium(IV): Contains titanium instead of tin.
Tetrakis(dimethylamido)hafnium(IV): Contains hafnium instead of tin.
Uniqueness
Tetrakis(dimethylamino)tin is unique due to its specific reactivity and volatility, making it particularly useful in applications requiring precise control over tin deposition and incorporation. Its ability to undergo various chemical reactions while maintaining stability under controlled conditions sets it apart from other organotin compounds.
生物活性
Tetrakis(dimethylamino)tin (TDMASn) is a chemical compound utilized primarily as a precursor in atomic layer deposition (ALD) for the fabrication of tin oxide films. Its biological activity, particularly in relation to its degradation products and the implications for electronic properties in devices such as solar cells, is an emerging area of research. This article explores the biological activity of TDMASn, detailing its chemical behavior, degradation pathways, and the resultant effects on material properties.
TDMASn is known for its role in producing high-quality tin oxide films through ALD. However, recent studies have highlighted significant degradation of TDMASn into bis(dimethylamido)tin(II) via a Sn-imine complex. This degradation occurs under typical processing conditions and has critical implications for the performance of devices utilizing these films.
Table 1: Degradation Pathways of TDMASn
Degradation Product | Chemical Formula | Impact on Properties |
---|---|---|
Tetrakis(dimethylamino)tin | TDMASn | Precursor for SnO2 films |
Bis(dimethylamido)tin(II) | (CH3)2N-Sn-N(CH3)2 | Increases electrical resistance; alters oxidation mechanisms |
Biological Activity and Implications
The biological activity of TDMASn is indirectly assessed through its impact on the electronic properties of tin oxide films. The degradation products significantly increase the electrical resistance of these films, which can lead to decreased efficiency in applications such as perovskite solar cells. The loss in power conversion efficiency is primarily attributed to charge transport and transfer losses caused by the different oxidation mechanisms of the degradation products.
Case Study: Impact on Solar Cell Efficiency
A recent study examined perovskite solar cells that incorporated tin oxide films derived from degraded TDMASn. The findings revealed:
- Power Conversion Efficiency (PCE) : A notable reduction in PCE was observed, with values dropping from 18% to 9% when using degraded materials.
- Charge Transport : Electrical characterization indicated that charge transport was severely hindered due to the higher resistance associated with the degradation products.
This case underscores the importance of maintaining the integrity of TDMASn during processing to ensure optimal performance in electronic applications.
Research Findings
Research has shown that while TDMASn is effective for ALD processes, its stability is a concern. Strategies to stabilize TDMASn or identify alternative precursors are being explored to mitigate these issues. The following findings summarize key research insights:
- Growth Kinetics : Despite degradation, growth kinetics and film morphology remained stable during ALD processes, suggesting that degradation does not affect the initial deposition rates.
- Electronic Properties : The electronic properties of films made from degraded TDMASn are significantly poorer, indicating a need for improved precursor stability.
- Film Quality : Films deposited at low temperatures (30-200 °C) maintain smooth surfaces but exhibit increased resistance when derived from degraded precursors.
Table 2: Summary of Research Findings
Parameter | Observation |
---|---|
Growth Rate | Stable despite precursor degradation |
Electrical Resistance | Increased due to degradation products |
Power Conversion Efficiency | Reduced significantly in solar cell applications |
特性
IUPAC Name |
N-methyl-N-[tris(dimethylamino)stannyl]methanamine | |
---|---|---|
Source | PubChem | |
URL | https://pubchem.ncbi.nlm.nih.gov | |
Description | Data deposited in or computed by PubChem | |
InChI |
InChI=1S/4C2H6N.Sn/c4*1-3-2;/h4*1-2H3;/q4*-1;+4 | |
Source | PubChem | |
URL | https://pubchem.ncbi.nlm.nih.gov | |
Description | Data deposited in or computed by PubChem | |
InChI Key |
WHXTVQNIFGXMSB-UHFFFAOYSA-N | |
Source | PubChem | |
URL | https://pubchem.ncbi.nlm.nih.gov | |
Description | Data deposited in or computed by PubChem | |
Canonical SMILES |
CN(C)[Sn](N(C)C)(N(C)C)N(C)C | |
Source | PubChem | |
URL | https://pubchem.ncbi.nlm.nih.gov | |
Description | Data deposited in or computed by PubChem | |
Molecular Formula |
C8H24N4Sn | |
Source | PubChem | |
URL | https://pubchem.ncbi.nlm.nih.gov | |
Description | Data deposited in or computed by PubChem | |
DSSTOX Substance ID |
DTXSID40147660 | |
Record name | Tetrakis(dimethylamino)tin | |
Source | EPA DSSTox | |
URL | https://comptox.epa.gov/dashboard/DTXSID40147660 | |
Description | DSSTox provides a high quality public chemistry resource for supporting improved predictive toxicology. | |
Molecular Weight |
295.01 g/mol | |
Source | PubChem | |
URL | https://pubchem.ncbi.nlm.nih.gov | |
Description | Data deposited in or computed by PubChem | |
CAS No. |
1066-77-9 | |
Record name | Tetrakis(dimethylamino)tin | |
Source | ChemIDplus | |
URL | https://pubchem.ncbi.nlm.nih.gov/substance/?source=chemidplus&sourceid=0001066779 | |
Description | ChemIDplus is a free, web search system that provides access to the structure and nomenclature authority files used for the identification of chemical substances cited in National Library of Medicine (NLM) databases, including the TOXNET system. | |
Record name | Tetrakis(dimethylamino)tin | |
Source | EPA DSSTox | |
URL | https://comptox.epa.gov/dashboard/DTXSID40147660 | |
Description | DSSTox provides a high quality public chemistry resource for supporting improved predictive toxicology. | |
Record name | dimethyl[tris(dimethylamino)stannyl]amine | |
Source | European Chemicals Agency (ECHA) | |
URL | https://echa.europa.eu/information-on-chemicals | |
Description | The European Chemicals Agency (ECHA) is an agency of the European Union which is the driving force among regulatory authorities in implementing the EU's groundbreaking chemicals legislation for the benefit of human health and the environment as well as for innovation and competitiveness. | |
Explanation | Use of the information, documents and data from the ECHA website is subject to the terms and conditions of this Legal Notice, and subject to other binding limitations provided for under applicable law, the information, documents and data made available on the ECHA website may be reproduced, distributed and/or used, totally or in part, for non-commercial purposes provided that ECHA is acknowledged as the source: "Source: European Chemicals Agency, http://echa.europa.eu/". Such acknowledgement must be included in each copy of the material. ECHA permits and encourages organisations and individuals to create links to the ECHA website under the following cumulative conditions: Links can only be made to webpages that provide a link to the Legal Notice page. | |
Retrosynthesis Analysis
AI-Powered Synthesis Planning: Our tool employs the Template_relevance Pistachio, Template_relevance Bkms_metabolic, Template_relevance Pistachio_ringbreaker, Template_relevance Reaxys, Template_relevance Reaxys_biocatalysis model, leveraging a vast database of chemical reactions to predict feasible synthetic routes.
One-Step Synthesis Focus: Specifically designed for one-step synthesis, it provides concise and direct routes for your target compounds, streamlining the synthesis process.
Accurate Predictions: Utilizing the extensive PISTACHIO, BKMS_METABOLIC, PISTACHIO_RINGBREAKER, REAXYS, REAXYS_BIOCATALYSIS database, our tool offers high-accuracy predictions, reflecting the latest in chemical research and data.
Strategy Settings
Precursor scoring | Relevance Heuristic |
---|---|
Min. plausibility | 0.01 |
Model | Template_relevance |
Template Set | Pistachio/Bkms_metabolic/Pistachio_ringbreaker/Reaxys/Reaxys_biocatalysis |
Top-N result to add to graph | 6 |
Feasible Synthetic Routes
Q1: How does Tetrakis(dimethylamido)tin(IV) enhance free radical photopolymerization under air, and what is the underlying chemical mechanism?
A1: Tetrakis(dimethylamido)tin(IV) acts as a coinitiator in Type II photoinitiating systems and an additive for Type I photoinitiators, significantly improving polymerization rates (Rp) under air []. This enhancement is attributed to its involvement in a bimolecular homolytic substitution (SH2) reaction.
試験管内研究製品の免責事項と情報
BenchChemで提示されるすべての記事および製品情報は、情報提供を目的としています。BenchChemで購入可能な製品は、生体外研究のために特別に設計されています。生体外研究は、ラテン語の "in glass" に由来し、生物体の外で行われる実験を指します。これらの製品は医薬品または薬として分類されておらず、FDAから任何の医療状態、病気、または疾患の予防、治療、または治癒のために承認されていません。これらの製品を人間または動物に体内に導入する形態は、法律により厳格に禁止されています。これらのガイドラインに従うことは、研究と実験において法的および倫理的な基準の遵守を確実にするために重要です。