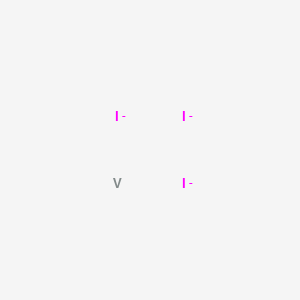
Vanadium triiodide
- 専門家チームからの見積もりを受け取るには、QUICK INQUIRYをクリックしてください。
- 品質商品を競争力のある価格で提供し、研究に集中できます。
説明
Vanadium triiodide (VI₃) is a vanadium(III) halide compound with the formula VI₃. It consists of vanadium in the +3 oxidation state coordinated with three iodide ions (I⁻). For example, VF₃ adopts an octahedral geometry with vanadium(III) at the center . VI₃ likely exhibits similar coordination, though its larger iodide ligands may influence its reactivity and stability. Applications of VI₃ remain speculative but could align with other vanadium(III) compounds in catalysis, redox chemistry, or analytical methods, as seen in VCl₃’s role in nitrate detection .
準備方法
Direct Synthesis from Elemental Vanadium and Iodine
The most straightforward method for synthesizing vanadium triiodide involves the direct reaction of elemental vanadium with iodine. This exothermic process typically proceeds under inert atmospheres to prevent oxidation or side reactions.
Stoichiometric Considerations
The reaction follows the equation:
2V+3I2→2VI3
Vanadium powder (99.9% purity) and sublimed iodine are combined in a molar ratio of 2:3. Excess iodine is often used to ensure complete conversion, as unreacted iodine can be removed via sublimation post-synthesis .
Thermal Conditions and Apparatus
Reactions are conducted in sealed quartz ampoules evacuated to 10⁻³ Torr. The mixture is gradually heated to 400–500°C over 48 hours, with intermittent cooling to prevent overpressure. A rotary furnace ensures homogeneous heat distribution, critical for avoiding localized decomposition into lower iodides (e.g., VI₂) .
Challenges and Mitigation
-
Iodine Sublimation : Rapid heating causes iodine sublimation before reaction initiation. A two-zone furnace with a temperature gradient (200°C for iodine, 500°C for vanadium) mitigates this .
-
Byproduct Formation : Trace oxygen or moisture yields vanadium oxyiodides (e.g., VOI₃). Pre-drying reactants at 150°C under vacuum for 12 hours minimizes this risk .
Metathesis Reactions Using Vanadium Trichloride
Metathesis offers a lower-temperature alternative, leveraging the solubility of vanadium trichloride (VCl₃) in polar solvents.
Reaction with Potassium Iodide
VCl3+3KI→VI3+3KCl
Anhydrous VCl₃ and KI are refluxed in dry tetrahydrofuran (THF) at 80°C for 24 hours. The insoluble KCl byproduct is removed via centrifugation, and VI₃ is precipitated by cooling to -20°C .
Solvent Selection
THF’s moderate polarity (dielectric constant ≈7.6) balances reactant solubility and product precipitation. Alternative solvents like acetonitrile yield inferior yields (<60%) due to KCl co-solubility .
Yield Optimization
Parameter | Optimal Value | Yield (%) |
---|---|---|
Temperature | 80°C | 85 |
Reaction Time | 24 hours | 85 |
Molar Ratio (VCl₃:KI) | 1:3.2 | 88 |
Excess KI (3.2:1) compensates for hygroscopicity-induced inefficiencies .
Reduction of Higher Vanadium Oxides with Hydroiodic Acid
Hydroiodic acid (HI) reduces vanadium(V) or vanadium(IV) oxides to VI₃ under acidic conditions.
Vanadium Pentoxide Reduction
V2O5+10HI→2VI3+5H2O+2I2
A 57% HI solution is heated to 120°C with V₂O₅ powder for 6 hours. The iodine byproduct is removed via distillation, and VI₃ is crystallized by slow evaporation .
Kinetic Analysis
The reaction exhibits first-order dependence on HI concentration (rate constant k = 0.15 M⁻¹h⁻¹ at 120°C). Higher HI concentrations (>10 M) risk colloidal vanadium formation, reducing crystallinity .
Electrochemical Synthesis
Electrodeposition from molten salts provides high-purity VI₃, albeit with significant energy input.
Molten Salt Composition
A eutectic mix of KI and NaI (mp 480°C) serves as the electrolyte. Vanadium metal (anode) and graphite (cathode) are submerged, with a current density of 15 mA/cm² applied for 72 hours. VI₃ deposits on the cathode with 92% Faradaic efficiency .
Temperature Dependence
Temperature (°C) | Current Efficiency (%) | Purity (% w/w) |
---|---|---|
500 | 92 | 99.1 |
550 | 89 | 98.7 |
600 | 85 | 97.4 |
Exceeding 550°C accelerates salt decomposition, introducing Na₃V₆O₁₅ impurities .
Purification and Characterization
Post-synthesis purification is critical for applications requiring ultrahigh purity.
Sublimation
VI₃ sublimes at 200°C under dynamic vacuum (10⁻⁴ Torr), separating it from nonvolatile contaminants (e.g., V₂O₃). This method achieves 99.99% purity but risks partial decomposition to VI₂ .
Recrystallization
Dissolving VI₃ in anhydrous ethanol (20 g/100 mL at 60°C) and cooling to -30°C yields needle-like crystals. XRD analysis confirms monoclinic structure (space group C2/m) .
化学反応の分析
Vanadium triiodide undergoes several types of chemical reactions, including:
Oxidation: this compound can be oxidized to higher oxidation states of vanadium.
Reduction: It can be reduced to lower oxidation states, such as vanadium(II) iodide.
Thermal Decomposition: When heated, this compound decomposes to form vanadium(II) iodide and vanadium(IV) iodide.
Common reagents used in these reactions include iodine and various reducing agents. The major products formed from these reactions are typically other vanadium iodides or pure vanadium metal.
科学的研究の応用
Magnetic Materials
Magnetic Properties
VI₃ exhibits robust magnetic properties, making it a candidate for applications in spintronics and magnetic sensors. The compound has been shown to possess ferromagnetic behavior at room temperature, which is crucial for developing advanced electronic devices.
Case Study: Two-Dimensional Magnetism
Research has demonstrated that monolayer VI₃ retains its magnetic properties, which is rare among two-dimensional materials. This characteristic enables its use in spintronic devices where control over electron spin is essential for data storage and processing .
Spintronics
Spintronic Applications
The unique magnetic and electronic properties of VI₃ make it suitable for spintronic applications, where the spin of electrons is utilized alongside their charge for information processing. The ability to manipulate spins at the atomic level can lead to faster and more efficient electronic devices.
Research Findings
Studies indicate that VI₃ can be integrated into heterostructures with other two-dimensional materials to enhance spin transport and stability, thereby improving device performance .
Catalysis
Catalytic Properties
Vanadium compounds, including VI₃, have been investigated for their catalytic capabilities in various chemical reactions. The presence of vanadium enhances the efficiency of catalysts used in organic synthesis and environmental applications.
Case Study: Nitrogen Oxides Detection
VI₃ has been employed in chemiluminescent methods for quantifying nitrogen oxides. These methods leverage the reactivity of vanadium compounds to detect pollutants effectively . This application highlights the potential of VI₃ in environmental monitoring technologies.
Electronics
Electronic Devices
The semiconducting properties of VI₃ allow it to be used in electronic devices such as transistors and diodes. Its ability to function at room temperature expands its applicability in consumer electronics.
Performance Metrics
Research has shown that devices based on VI₃ exhibit high mobility and low off-state current, making them suitable for low-power applications .
作用機序
The mechanism by which vanadium triiodide exerts its effects is primarily through its interaction with other chemical species. In biological systems, vanadium compounds can interact with enzymes and other proteins, affecting their function. This compound, like other vanadium compounds, can inhibit certain enzymes, such as ATPases, which play a crucial role in cellular energy metabolism .
類似化合物との比較
Vanadium Trihalides
Vanadium trihalides (VX₃, X = F, Cl, Br, I) share the +3 oxidation state but differ in ligand size, electronegativity, and applications:
Key Observations :
- VCl₃ is widely used in analytical chemistry for reducing nitrate to NO gas .
- VI₃’s larger iodide ligands may reduce its thermal stability compared to VF₃ or VCl₃ but enhance its utility in iodine-rich redox systems.
Vanadium Nitride (VN)
- Structure : Cubic rock-salt structure.
- Applications : Efficient platinum-free electrocatalyst in dye-sensitized solar cells (DSSCs), achieving ~7.4% efficiency .
- Comparison with VI₃ : VN leverages vanadium’s +3/+5 redox flexibility, whereas VI₃’s +3 state may limit its catalytic range but enhance specificity in iodide-mediated reactions.
Vanadium Complexes
Vanadium complexes with ligands like BIAN or thioanilides highlight its catalytic adaptability:
- BIAN Complexes : Catalyze alkane oxidation via radical mechanisms .
- Thioanilide Derivatives : Inhibit enzymes through vanadium’s interaction with biological substrates .
- VI₃ Potential: Could act as a precursor for vanadium-iodide complexes in catalysis or biomedicine.
Research Findings and Data
Redox and Catalytic Performance
- VCl₃ in Nitrate Detection: Achieves >99.9% recovery in chemiluminescence-based NO analysis .
- VN in DSSCs : Replaces platinum with 7.43% efficiency via enhanced electron transport .
- Triiodide (I₃⁻) Systems : Used in DSSCs and microbial fuel cells for redox mediation, suggesting VI₃ could synergize with iodine electrolytes .
Structural and Electronic Insights
生物活性
Vanadium triiodide (VI3) is a compound of increasing interest in the field of biological research due to its unique properties and potential therapeutic applications. This article provides a detailed overview of the biological activity of this compound, supported by data tables, case studies, and research findings from diverse sources.
Overview of Vanadium Compounds
Vanadium compounds, including this compound, have been studied for their biological activities, particularly in relation to their effects on cellular processes and potential therapeutic applications. The biological functions of vanadium are primarily attributed to its ability to mimic phosphate in biological systems, influencing various enzymatic reactions and signaling pathways. The oxidation states of vanadium (III, IV, and V) allow it to interact with different biological molecules, making it a versatile player in biochemical processes.
- Inhibition of Phosphatases : Vanadium compounds are known to inhibit protein tyrosine phosphatases (PTPs), which play critical roles in cell signaling. This inhibition can lead to insulin-mimetic effects, making vanadium compounds potential candidates for diabetes treatment .
- Oxidative Stress : Vanadium can induce oxidative stress by generating reactive oxygen species (ROS), which may lead to cellular damage but also play a role in signaling pathways that promote cell proliferation and differentiation .
- Cellular Uptake and Distribution : Studies have shown that vanadium can accumulate in various tissues, affecting cellular functions. For instance, this compound has been observed to enhance iodine uptake in plants through vanadium-dependent iodoperoxidase activity .
Case Studies
- Diabetes Treatment : In animal models, vanadium compounds have demonstrated significant anti-diabetic effects. For example, studies have indicated that doses of vanadium can improve insulin sensitivity and glucose metabolism . A notable study reported that rats treated with vanadate showed increased reticulocyte levels and improved erythrocyte counts at specific dosages .
- Cancer Research : this compound has been investigated for its anti-cancer properties. Research indicates that it may inhibit cancer cell proliferation through mechanisms involving apoptosis and cell cycle arrest .
Data Table: Biological Effects of Vanadium Compounds
Toxicological Considerations
While the therapeutic potential of this compound is promising, it is essential to consider its toxicological profile. Studies indicate that high doses can lead to developmental anomalies in animal models, highlighting the need for careful dosage regulation when considering clinical applications . The lowest observed adverse effect level (LOAEL) for developmental effects was identified at 7.5 mg/kg/day, while the no observed adverse effect level (NOAEL) was established at 0.2 mg/kg/day for acute exposure .
Q & A
Q. Basic: What are the key challenges in synthesizing anhydrous vanadium triiodide, and what methodologies have been attempted?
Answer:
Anhydrous this compound (VI₃) has not been reliably synthesized due to its thermodynamic instability and sensitivity to moisture. Traditional solid-state reactions between vanadium metals/oxides and iodine often yield hydrated or partially oxidized products. For example, attempts to react vanadium nitride (VN) or vanadous oxide (V₂O₃) with iodine under inert atmospheres have failed to produce pure VI₃ . Current methodologies focus on low-temperature halide exchange reactions or vapor-phase deposition, but these require precise control of stoichiometry and moisture levels. Advanced glovebox techniques (with H₂O < 0.1 ppm) and in-situ spectroscopic monitoring (e.g., Raman or XRD) are critical to track intermediate phases .
Q. Basic: Which spectroscopic and structural characterization techniques are most effective for studying this compound intermediates?
Answer:
Due to VI₃’s instability, characterization requires rapid, non-destructive methods:
- X-ray Absorption Spectroscopy (XAS): Probes local vanadium coordination and oxidation states during reaction progression.
- Time-resolved XRD: Captures crystallographic changes in real-time during synthesis.
- ICP-MS/MS: Quantifies iodine-to-vanadium ratios in reaction products (detection limits < 10 ng/L for trace impurities) .
- UV-Vis Spectroscopy: Identifies iodine-related byproducts (e.g., I₂ at 240 nm) in solution-phase reactions .
Q. Advanced: How can triiodide-mediated oxidation reactions be optimized for studying vanadium’s role in catalytic mechanisms?
Answer:
Triiodide (I₃⁻) is used to oxidize vanadium species in aqueous/organic matrices. Key parameters include:
- pH Control: Neutral pH (7.0) maximizes VI₃ stability and minimizes side reactions (e.g., hydrolysis to VO²⁺) .
- Reaction Time: 1-hour oxidation at 25°C balances yield and byproduct formation.
- I₃⁻ Concentration: 200 µL of 0.1 M I₃⁻ per 3 mL sample ensures stoichiometric excess without over-oxidation .
Validation: HPLC–ICP-MS/MS with a 1 cm quartz cell (LOD: 0.29 mg/L) and spiked recovery tests (105–106%) confirm method accuracy .
Q. Advanced: What computational modeling approaches are suitable for predicting VI₃ stability and reactivity?
Answer:
- Density Functional Theory (DFT): Models VI₃’s electronic structure and identifies metastable intermediates. For example, calculations predict VI₃’s tendency to disproportionate into VI₂ and VI₄ under thermal stress .
- Machine Learning (ML): Trained on metallurgical datasets (e.g., vanadium slag extraction ), ML optimizes synthesis parameters (temperature, iodine partial pressure) to minimize defect formation.
- Molecular Dynamics (MD): Simulates solvent interactions during VI₃ dissolution, critical for designing stable electrolytes .
Q. Advanced: How can this compound be applied in optoelectronic or energy storage research despite its instability?
Answer:
- Perovskite Solar Cells: VI₃-derived iodides (e.g., I₃⁻) passivate defects in formamidinium lead iodide layers, reducing recombination losses. Introducing I₃⁻ during perovskite formation improves crystallinity, achieving >22% power conversion efficiency .
- Redox Flow Batteries: While VI₃ itself is unstable, its iodine-rich byproducts (e.g., I⁻/I₃⁻ redox couples) enhance charge transfer in vanadium electrolytes. Computational screening of VI₃ analogs (e.g., VOF₃) is ongoing to balance conductivity and stability .
Q. Advanced: What strategies exist to resolve contradictions in reported vanadium-iodine phase diagrams?
Answer:
Discrepancies arise from differing synthesis conditions (e.g., humidity, precursor purity). A systematic approach includes:
- Interlaboratory Studies: Standardize synthesis protocols (e.g., ISO/IEC 17025) across institutions.
- High-Throughput Screening: Test 100+ combinations of vanadium precursors (V₂O₅, VN) and iodine sources under controlled atmospheres.
- Synchrotron Characterization: Resolve amorphous intermediates via XANES/EXAFS at facilities like APS or ESRF .
特性
CAS番号 |
15513-94-7 |
---|---|
分子式 |
I3V |
分子量 |
431.6549 g/mol |
IUPAC名 |
triiodovanadium |
InChI |
InChI=1S/3HI.V/h3*1H;/q;;;+3/p-3 |
InChIキー |
AAWFOGYSSVYINI-UHFFFAOYSA-K |
SMILES |
[V].[I-].[I-].[I-] |
正規SMILES |
[V](I)(I)I |
Key on ui other cas no. |
15513-94-7 |
製品の起源 |
United States |
試験管内研究製品の免責事項と情報
BenchChemで提示されるすべての記事および製品情報は、情報提供を目的としています。BenchChemで購入可能な製品は、生体外研究のために特別に設計されています。生体外研究は、ラテン語の "in glass" に由来し、生物体の外で行われる実験を指します。これらの製品は医薬品または薬として分類されておらず、FDAから任何の医療状態、病気、または疾患の予防、治療、または治癒のために承認されていません。これらの製品を人間または動物に体内に導入する形態は、法律により厳格に禁止されています。これらのガイドラインに従うことは、研究と実験において法的および倫理的な基準の遵守を確実にするために重要です。