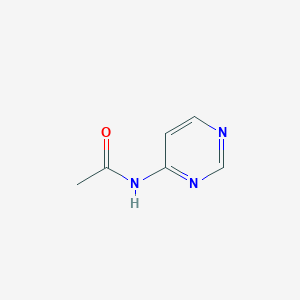
N-(ピリミジン-4-イル)アセトアミド
概要
説明
N-(Pyrimidin-4-yl)acetamide is an organic compound with the molecular formula C6H7N3O. It belongs to the class of pyrimidine derivatives, which are known for their diverse biological activities and applications in medicinal chemistry . The compound features a pyrimidine ring substituted with an acetamide group at the 4-position, making it a valuable scaffold in drug discovery and development.
科学的研究の応用
N-(Pyrimidin-4-yl)acetamide has a wide range of applications in scientific research, including:
作用機序
Target of Action
N-(Pyrimidin-4-yl)acetamide is a pyrimidine derivative . Pyrimidine derivatives have been found to target Cyclin-dependent kinase 2 (CDK2) . CDK2 is a crucial enzyme involved in cell cycle regulation, and its inhibition can lead to cell cycle arrest .
Mode of Action
Pyrimidine derivatives are known to inhibit their targets by competing with atp for binding to the kinase domain . This prevents the phosphorylation of substrate proteins, thereby inhibiting the activity of the target enzyme .
Biochemical Pathways
The inhibition of CDK2 affects the cell cycle, particularly the transition from the G1 phase to the S phase . This can lead to cell cycle arrest and potentially induce apoptosis . Furthermore, pyrimidines have been reported to inhibit the expression and activities of certain vital inflammatory mediators such as prostaglandin E2, inducible nitric oxide synthase, tumor necrosis factor-α, nuclear factor κB, leukotrienes, and some interleukins .
Pharmacokinetics
Related pyrimidine derivatives have shown dose- and time-dependent pharmacokinetics in human subjects . The absorption, distribution, metabolism, and excretion (ADME) properties of these compounds can significantly impact their bioavailability and efficacy .
Result of Action
The inhibition of CDK2 by N-(Pyrimidin-4-yl)acetamide can lead to cell cycle arrest, potentially inducing apoptosis . This can result in the death of rapidly dividing cells, such as cancer cells . Additionally, the inhibition of inflammatory mediators can lead to anti-inflammatory effects .
Action Environment
The action, efficacy, and stability of N-(Pyrimidin-4-yl)acetamide can be influenced by various environmental factors. For instance, the pH of the environment can affect the ionization state of the compound, influencing its absorption and distribution . Additionally, the presence of other substances, such as food or other drugs, can impact the metabolism and excretion of the compound .
Safety and Hazards
準備方法
Synthetic Routes and Reaction Conditions: N-(Pyrimidin-4-yl)acetamide can be synthesized through various methods. One common approach involves the reaction of pyrimidine-4-carboxylic acid with acetic anhydride in the presence of a catalyst such as sulfuric acid. The reaction typically proceeds under reflux conditions, yielding N-(Pyrimidin-4-yl)acetamide after purification .
Industrial Production Methods: In an industrial setting, the synthesis of N-(Pyrimidin-4-yl)acetamide may involve the use of continuous flow reactors to optimize reaction conditions and improve yield. The process may include steps such as solvent extraction, crystallization, and recrystallization to ensure high purity of the final product .
化学反応の分析
Types of Reactions: N-(Pyrimidin-4-yl)acetamide undergoes various chemical reactions, including:
Common Reagents and Conditions:
Oxidation: Potassium permanganate in acidic medium.
Reduction: Lithium aluminum hydride in anhydrous ether.
Substitution: Alkyl halides in the presence of a base such as sodium hydroxide.
Major Products:
Oxidation: Pyrimidine-4-carboxylic acid derivatives.
Reduction: Pyrimidine-4-methanol derivatives.
Substitution: Various substituted pyrimidine derivatives.
類似化合物との比較
N-(Pyridin-2-yl)acetamide: This compound features a pyridine ring instead of a pyrimidine ring and exhibits similar biological activities.
N-(Pyrimidin-2-yl)acetamide: Similar to N-(Pyrimidin-4-yl)acetamide but with the acetamide group at the 2-position, affecting its chemical reactivity and biological properties.
Uniqueness: N-(Pyrimidin-4-yl)acetamide is unique due to its specific substitution pattern, which influences its chemical reactivity and interaction with biological targets. This makes it a valuable scaffold for the development of novel therapeutic agents with distinct pharmacological profiles .
特性
IUPAC Name |
N-pyrimidin-4-ylacetamide | |
---|---|---|
Source | PubChem | |
URL | https://pubchem.ncbi.nlm.nih.gov | |
Description | Data deposited in or computed by PubChem | |
InChI |
InChI=1S/C6H7N3O/c1-5(10)9-6-2-3-7-4-8-6/h2-4H,1H3,(H,7,8,9,10) | |
Source | PubChem | |
URL | https://pubchem.ncbi.nlm.nih.gov | |
Description | Data deposited in or computed by PubChem | |
InChI Key |
MVCZKPMTGFFULA-UHFFFAOYSA-N | |
Source | PubChem | |
URL | https://pubchem.ncbi.nlm.nih.gov | |
Description | Data deposited in or computed by PubChem | |
Canonical SMILES |
CC(=O)NC1=NC=NC=C1 | |
Source | PubChem | |
URL | https://pubchem.ncbi.nlm.nih.gov | |
Description | Data deposited in or computed by PubChem | |
Molecular Formula |
C6H7N3O | |
Source | PubChem | |
URL | https://pubchem.ncbi.nlm.nih.gov | |
Description | Data deposited in or computed by PubChem | |
DSSTOX Substance ID |
DTXSID10311851 | |
Record name | 4-acetamidopyrimidine | |
Source | EPA DSSTox | |
URL | https://comptox.epa.gov/dashboard/DTXSID10311851 | |
Description | DSSTox provides a high quality public chemistry resource for supporting improved predictive toxicology. | |
Molecular Weight |
137.14 g/mol | |
Source | PubChem | |
URL | https://pubchem.ncbi.nlm.nih.gov | |
Description | Data deposited in or computed by PubChem | |
CAS No. |
16166-22-6 | |
Record name | 16166-22-6 | |
Source | DTP/NCI | |
URL | https://dtp.cancer.gov/dtpstandard/servlet/dwindex?searchtype=NSC&outputformat=html&searchlist=246063 | |
Description | The NCI Development Therapeutics Program (DTP) provides services and resources to the academic and private-sector research communities worldwide to facilitate the discovery and development of new cancer therapeutic agents. | |
Explanation | Unless otherwise indicated, all text within NCI products is free of copyright and may be reused without our permission. Credit the National Cancer Institute as the source. | |
Record name | 4-acetamidopyrimidine | |
Source | EPA DSSTox | |
URL | https://comptox.epa.gov/dashboard/DTXSID10311851 | |
Description | DSSTox provides a high quality public chemistry resource for supporting improved predictive toxicology. | |
Synthesis routes and methods
Procedure details
Retrosynthesis Analysis
AI-Powered Synthesis Planning: Our tool employs the Template_relevance Pistachio, Template_relevance Bkms_metabolic, Template_relevance Pistachio_ringbreaker, Template_relevance Reaxys, Template_relevance Reaxys_biocatalysis model, leveraging a vast database of chemical reactions to predict feasible synthetic routes.
One-Step Synthesis Focus: Specifically designed for one-step synthesis, it provides concise and direct routes for your target compounds, streamlining the synthesis process.
Accurate Predictions: Utilizing the extensive PISTACHIO, BKMS_METABOLIC, PISTACHIO_RINGBREAKER, REAXYS, REAXYS_BIOCATALYSIS database, our tool offers high-accuracy predictions, reflecting the latest in chemical research and data.
Strategy Settings
Precursor scoring | Relevance Heuristic |
---|---|
Min. plausibility | 0.01 |
Model | Template_relevance |
Template Set | Pistachio/Bkms_metabolic/Pistachio_ringbreaker/Reaxys/Reaxys_biocatalysis |
Top-N result to add to graph | 6 |
Feasible Synthetic Routes
Q1: How does N-(pyrimidin-4-yl)acetamide interact with its target and what are the downstream effects?
A1: N-(pyrimidin-4-yl)acetamides, specifically the 2-amino-N-pyrimidin-4-yl acetamides discussed in the research, act as antagonists of the adenosine A2A receptor (A2AR). [] While the exact binding mechanism isn't detailed in the provided abstract, these antagonists likely bind to the A2AR, preventing the endogenous ligand, adenosine, from binding and activating the receptor. This blockage can have various downstream effects, particularly within the central nervous system. Inhibiting A2AR signaling can increase dopamine release and neurotransmission, making these compounds potentially useful for treating neurological disorders like Parkinson's disease. []
Q2: What is known about the structure-activity relationship (SAR) of N-(pyrimidin-4-yl)acetamides and how do modifications impact their activity and selectivity for the A2A receptor?
A2: The research focuses on enhancing the aqueous solubility of N-(pyrimidin-4-yl)acetamides while maintaining their potency and selectivity as A2AR antagonists. [] The abstract highlights the exploration of 2-amino-N-pyrimidin-4-yl acetamides as a promising structural variation. Although specific modifications aren't detailed, the study likely investigates how different substituents on the pyrimidine ring and the acetamide nitrogen affect the compound's interaction with the A2AR binding site, impacting its potency and selectivity. The research also notes a difference in potency between rat and human A2A receptors, suggesting further SAR studies could optimize compounds for specific species. []
試験管内研究製品の免責事項と情報
BenchChemで提示されるすべての記事および製品情報は、情報提供を目的としています。BenchChemで購入可能な製品は、生体外研究のために特別に設計されています。生体外研究は、ラテン語の "in glass" に由来し、生物体の外で行われる実験を指します。これらの製品は医薬品または薬として分類されておらず、FDAから任何の医療状態、病気、または疾患の予防、治療、または治癒のために承認されていません。これらの製品を人間または動物に体内に導入する形態は、法律により厳格に禁止されています。これらのガイドラインに従うことは、研究と実験において法的および倫理的な基準の遵守を確実にするために重要です。