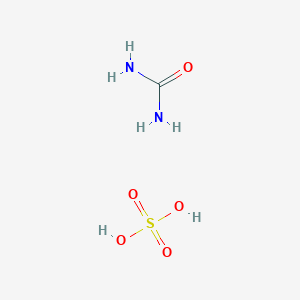
Urea sulfate
概要
説明
Urea sulfate (CO(NH₂)₂·H₂SO₄) is a compound formed by the reaction of urea with sulfuric acid. It is a stable crystalline salt that combines the nitrogen-rich urea molecule with sulfate ions. Urea is widely used as a nitrogen fertilizer, while sulfates (e.g., ammonium sulfate, copper sulfate) are critical in agriculture, industrial processes, and environmental chemistry. This compound’s dual composition suggests applications in controlled nitrogen-sulfur release, soil conditioning, and chemical synthesis .
準備方法
Conventional Solution Reaction and Granulation Process
The most widely documented method for urea sulfate preparation involves the controlled reaction of urea and sulfuric acid under elevated temperatures, followed by granulation and drying. This approach, detailed in patent CN102358726A , emphasizes precision in temperature control and reactant ratios to optimize yield and product stability.
Reaction Conditions and Stoichiometry
This compound synthesis occurs in a reactive tank where preheated urea and sulfuric acid solutions are mixed at mass ratios ranging from 11.83:1 to 2:1 (urea:sulfuric acid). The process mandates:
-
Urea solution preheating : 75°C–118°C using 150°C steam at 0.5 MPa pressure.
-
Sulfuric acid preheating : 60°C–100°C to ensure optimal viscosity for mixing.
At these conditions, the reaction proceeds exothermically, forming a this compound slurry. The nitrogen-to-sulfur (N:S) mass ratio in the final product varies between 10:1 and 1:1 , depending on the initial urea-sulfuric acid ratio .
Granulation and Post-Treatment
The slurry is atomized into a rotary drum granulator, where it adheres to seed particles, forming granules of 1.18–3.35 mm . Key post-treatment steps include:
-
Drying : Utilization of calcium oxide (CaO) or calcium hydroxide (Ca(OH)₂) to absorb residual moisture and sulfur dioxide (SO₂) emissions.
-
Cooling : Revolution coolers reduce granule temperature to prevent caking.
-
Sieving : Particles outside the target size range are recycled, ensuring >95% yield efficiency .
Table 1: Process Parameters and Product Characteristics in this compound Synthesis
This method minimizes wastewater generation by recycling tail gases through scrubbing systems and repurposing steam condensate .
High-Shear Mixing for Enhanced Crystallization
While primarily applied to urea calcium sulfate (UCS) production, high-shear mixing technology offers insights into optimizing this compound’s crystalline structure. A continuous process described in EP4183764B1 employs shear rates exceeding 50,000/s to accelerate reaction kinetics and improve particle uniformity. Although tailored for UCS, this method’s principles—such as rapid slurry homogenization and controlled water removal—are adaptable to this compound synthesis.
Key Adaptations for this compound
-
Shear-induced crystallization : High shear forces reduce particle size distribution, enhancing nutrient release rates.
-
Water removal : Vacuum evaporation or spray drying achieves <1% moisture content, critical for storage stability .
Pipe Reactor Synthesis for Scalable Production
Industrial-scale urea ammonium sulfate (UAS) production using pipe reactors, as outlined in EP1861335B1 , provides a template for this compound manufacturing. The reactor facilitates:
-
Continuous flow reactions : Urea and sulfuric acid are introduced at opposing ends, ensuring thorough mixing.
-
In-line neutralization : Acidic vapors are scrubbed using ammonia, reducing emissions .
While UAS differs chemically from this compound, the reactor design—particularly its ability to handle corrosive media and high temperatures—is directly applicable.
Impact of Process Parameters on Product Efficacy
Temperature and Stoichiometry
Higher preheating temperatures (e.g., 117°C) favor the formation of needle-like crystals with elevated N:S ratios (10:1), whereas lower temperatures (75°C–91.5°C) produce prismatic crystals with balanced N:S profiles (3:1–1:1) . These structural differences influence dissolution rates and nutrient availability, as demonstrated in agronomic trials where this compound with N:S = 3:1 increased maize yields by 23.07% compared to conventional urea .
Additives and Impurity Control
Patent data specifies strict limits for byproducts:
-
Biuret : ≤1.5% to prevent phytotoxicity.
-
Free acid : ≤0.05% to avoid soil acidification .
Incorporating magnesium (Mg) and micronutrients (Zn, Cu) during granulation enhances the fertilizer’s secondary nutrient content without affecting reaction efficiency .
Environmental and Economic Considerations
The described methods prioritize sustainability:
化学反応の分析
Thermal Decomposition and Byproduct Formation
At elevated temperatures (>160°C), urea sulfate undergoes thermolysis to form biuret (NH₂CONHCONH₂) and ammonia :
This reaction is influenced by residual sulfuric acid, which catalyzes further decomposition into cyanuric acid and melamine derivatives under extreme conditions .
Key Byproducts Observed :
-
Biuret : ≤1.5% mass fraction
-
Free acid (H₂SO₄) : ≤0.2%
-
Trace metals : Mg, Zn, Cu (≥2% moderate elements, ≥0.02% micronutrients)
Reaction Kinetics and Optimization
A study modeling urea-sulfuric acid reactions identified critical parameters for maximizing conversion :
Table 2: Reaction Kinetics Under Varied Conditions
Acid:Urea Ratio | Reaction Rate (×10⁻⁵ s⁻¹) | Time for Full Conversion | Adiabatic Temp Rise (°C) |
---|---|---|---|
1.5:1 | 1.0 | ~10 hours | 12–15 |
3:1 | 2.5 | ~6 hours | 18–22 |
10:1 | 4.8 | ~3 hours | 25–30 |
Higher acid ratios accelerate urea conversion but increase corrosive byproducts (e.g., SO₂). Optimal conditions balance efficiency and safety .
pH-Dependent Behavior
This compound solutions exhibit pH-dependent dissociation:
At pH > 8.6 (Embodiment 1), the compound stabilizes as a weak base, while acidic conditions (pH 6.5–7.2) promote ammonium sulfate formation .
科学的研究の応用
Urea sulfate has a wide range of applications in scientific research, including:
Agriculture: Used as a fertilizer to provide both nitrogen and sulfur to plants, enhancing growth and yield.
Chemistry: Employed as a reagent in various chemical reactions, including the synthesis of other compounds.
Biology: Utilized in studies involving nitrogen metabolism and sulfur assimilation in plants.
Medicine: Investigated for its potential use in drug formulations and as a component in certain medical treatments.
Industry: Used in the production of resins, plastics, and other industrial materials
作用機序
The mechanism of action of urea sulfate primarily involves its ability to release nitrogen and sulfur upon decomposition or hydrolysis. In plants, the nitrogen from this compound is converted into ammonium and nitrate, which are essential nutrients for plant growth. The sulfur is assimilated into amino acids and proteins, playing a crucial role in various metabolic processes. The molecular targets include enzymes involved in nitrogen and sulfur metabolism, such as urease and sulfur reductase .
類似化合物との比較
Comparative Analysis with Similar Compounds
Chemical Structure and Solubility
- Urea Sulfate : Likely exists as a salt with urea protonated by sulfuric acid. Its structure enables simultaneous release of urea (a neutral molecule) and sulfate ions (SO₄²⁻) in solution.
- Ammonium Sulfate ((NH₄)₂SO₄): A fully ionic salt with two ammonium cations and one sulfate anion. Highly water-soluble (70.6 g/100 mL at 0°C) and dissociates into NH₄⁺ and SO₄²⁻, contributing to soil acidification .
- Copper(II) Sulfate (CuSO₄): Ionic compound with Cu²⁺ and SO₄²⁻. Solubility in water is moderate (32 g/100 mL at 20°C) and used as a fungicide and in industrial adhesives .
- Urea (CO(NH₂)₂): Neutral organic compound with high solubility (108 g/100 mL at 20°C).
Key Difference : this compound combines organic and ionic components, whereas ammonium and copper sulfates are purely ionic. This hybrid nature may influence solubility and reactivity in environmental or industrial contexts.
Q & A
Basic Research Questions
Q. How can researchers optimize the synthesis of urea sulfate for high-purity yields in laboratory settings?
Methodological Answer:
- Step 1: Use stoichiometric ratios of urea and sulfuric acid (typically 1:1 molar ratio) under controlled temperature (e.g., 40–60°C) to minimize side reactions .
- Step 2: Employ recrystallization in ethanol or water to purify the product, monitoring pH to avoid decomposition .
- Step 3: Validate purity via elemental analysis (C, H, N, S) and compare results with theoretical values. Cross-reference with IR spectroscopy to confirm functional groups (e.g., sulfate peaks at ~1100 cm⁻¹) .
Q. What spectroscopic techniques are most effective for characterizing this compound’s molecular structure and purity?
Methodological Answer:
- IR Spectroscopy: Identify sulfate (S=O) and urea (N-H) functional groups. Peaks at 1680 cm⁻¹ (urea C=O) and 1100 cm⁻¹ (sulfate) confirm structure .
- NMR: Use ¹³C NMR to resolve urea carbon environments and detect impurities (e.g., unreacted urea at ~160 ppm) .
- XRD: Compare diffraction patterns with crystallographic databases (e.g., ICDD) to confirm phase purity .
Advanced Research Questions
Q. How should experimental designs be structured to evaluate this compound’s efficacy as a nitrogen source in plant growth studies compared to other fertilizers?
Methodological Answer:
- Design: Use randomized controlled trials (RCTs) with variables like soil pH, application rates, and crop type (e.g., sunflower in acidic soils ).
- Controls: Include ammonium sulfate and urea as comparative treatments. Measure achene yield, leaf N concentration, and soil residual N post-harvest .
- Data Analysis: Apply ANOVA to identify significant differences (p < 0.05) and use Tukey’s HSD for post-hoc comparisons. Address confounding variables (e.g., soil organic matter) via regression modeling .
Q. What methodologies are recommended for resolving contradictions in reported thermal stability data of this compound under varying environmental conditions?
Methodological Answer:
- Controlled Decomposition Studies: Conduct thermogravimetric analysis (TGA) at incremental heating rates (e.g., 5–20°C/min) to isolate decomposition kinetics .
- Environmental Simulation: Replicate humidity and temperature conditions from conflicting studies (e.g., 30–80% RH, 25–50°C) to identify stability thresholds .
- Data Reconciliation: Use multivariate analysis to isolate factors (e.g., impurities, crystallinity) affecting stability. Cross-validate with FTIR to detect intermediate degradation products .
Q. How can researchers validate the reproducibility of this compound’s reported solubility profile across different solvent systems?
Methodological Answer:
- Step 1: Standardize solvent preparation (e.g., deionized water, ethanol purity ≥99.5%) and temperature control (±0.1°C) .
- Step 2: Use gravimetric analysis for solubility quantification. Saturate solvents, filter undissolved solids, and evaporate to constant weight .
- Step 3: Compare results with literature using Bland-Altman plots to assess systematic biases. Report confidence intervals for solubility values .
Q. Data Contradiction Analysis
Q. How should researchers address discrepancies in this compound’s hygroscopicity reported across studies?
Methodological Answer:
- Hypothesis Testing: Attribute discrepancies to environmental factors (e.g., relative humidity, storage duration) or measurement techniques (dynamic vs. static gravimetry) .
- Experimental Replication: Repeat studies under identical conditions (e.g., 25°C, 60% RH) using Karl Fischer titration for precise moisture quantification .
- Meta-Analysis: Aggregate data from multiple studies, applying random-effects models to quantify heterogeneity and identify outliers .
Q. Best Practices for Literature Review & Data Validation
- Critical Appraisal: Evaluate studies for methodological rigor (e.g., sample size, controls) and conflict-of-interest disclosures .
- Data Triangulation: Cross-reference experimental findings with computational models (e.g., DFT calculations for thermal stability) .
- Replicability: Document detailed protocols (e.g., reaction times, equipment calibration) to enable independent verification .
特性
CAS番号 |
19082-42-9 |
---|---|
分子式 |
CH6N2O5S |
分子量 |
158.14 g/mol |
IUPAC名 |
carbamoylazanium;hydrogen sulfate |
InChI |
InChI=1S/CH4N2O.H2O4S/c2-1(3)4;1-5(2,3)4/h(H4,2,3,4);(H2,1,2,3,4) |
InChIキー |
SSBRSHIQIANGKS-UHFFFAOYSA-N |
SMILES |
C(=O)(N)N.OS(=O)(=O)O |
正規SMILES |
C(=O)([NH3+])N.OS(=O)(=O)[O-] |
Key on ui other cas no. |
21351-39-3 19082-42-9 |
物理的記述 |
Liquid |
ピクトグラム |
Corrosive |
関連するCAS |
17103-31-0 |
製品の起源 |
United States |
Synthesis routes and methods
Procedure details
試験管内研究製品の免責事項と情報
BenchChemで提示されるすべての記事および製品情報は、情報提供を目的としています。BenchChemで購入可能な製品は、生体外研究のために特別に設計されています。生体外研究は、ラテン語の "in glass" に由来し、生物体の外で行われる実験を指します。これらの製品は医薬品または薬として分類されておらず、FDAから任何の医療状態、病気、または疾患の予防、治療、または治癒のために承認されていません。これらの製品を人間または動物に体内に導入する形態は、法律により厳格に禁止されています。これらのガイドラインに従うことは、研究と実験において法的および倫理的な基準の遵守を確実にするために重要です。