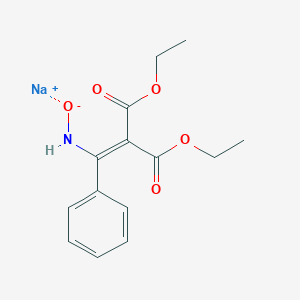
BROMOXYNILPHENOL
説明
BROMOXYNILPHENOL is a complex organic compound that belongs to the family of oximes. Oximes are characterized by the presence of the functional group -C=N-OH, which is derived from the reaction of hydroxylamine with aldehydes or ketones. This particular compound is notable for its unique structure, which includes both ethoxy and oxime groups, making it a subject of interest in various fields of scientific research.
準備方法
Synthetic Routes and Reaction Conditions
The synthesis of BROMOXYNILPHENOL typically involves the following steps:
Formation of the oxime group: This is achieved by reacting hydroxylamine with an appropriate aldehyde or ketone. In this case, the starting material is likely a derivative of cinnamic acid.
Esterification: The carboxylic acid group of the cinnamic acid derivative is esterified with ethanol to form the ethyl ester.
Formation of the sodium salt: The final step involves neutralizing the compound with sodium hydroxide to form the sodium salt.
Industrial Production Methods
Industrial production of this compound would follow similar steps but on a larger scale, with optimizations for yield and purity. This might include the use of continuous flow reactors for the esterification and ethoxylation steps, as well as advanced purification techniques such as recrystallization and chromatography.
化学反応の分析
Hydrolysis and Ester Degradation
Bromoxynil phenol is primarily formed via hydrolysis of bromoxynil esters such as bromoxynil octanoate and heptanoate, common active ingredients in commercial herbicides.
-
Mechanism : Esterases or abiotic hydrolysis cleave the ester bond, releasing bromoxynil phenol.
-
Environmental Impact : Esters exhibit low volatility (Henry’s law constant: 5.3×10⁻⁴ Pa·m³/mol) and minimal groundwater contamination risk due to rapid degradation .
Anaerobic Reductive Debromination
Microbial degradation under anaerobic conditions is a major detoxification pathway:
text3,5-Dibromo-4-hydroxybenzonitrile → 4-Cyanophenol + 2Br⁻
Organism | Debromination Efficiency | Doubling Time | Stoichiometry | Sources |
---|---|---|---|---|
Desulfitobacterium chlororespirans | 100% (35–75 μM substrate) | 18.4 ± 5.2 hours | 1:1 conversion to 4-cyanophenol |
-
Key Findings :
Oxidative Degradation
Bromoxynil phenol undergoes oxidation in aerobic environments, forming hydroxylated and nitrile-derived metabolites:
-
Mechanism : Cytochrome P450 enzymes and hydroxyl radicals mediate nitrile group oxidation and aryl dehalogenation .
Photodegradation
Sunlight accelerates degradation in surface waters:
Parameter | Value | Impact | Sources |
---|---|---|---|
Photolytic half-life | 0.07 days (bromoxynil phenol) | Rapid breakdown under UV light | |
Major products | CO₂, halogenated byproducts | Mineralization dominates in clear water |
-
Limitation : Limited data on intermediate photoproducts in natural systems.
Plant Metabolism:
-
Bromoxynil esters are rapidly hydrolyzed to bromoxynil phenol in crops (e.g., wheat, onion) .
-
Further conjugation with sugars (e.g., glucuronides) enhances water solubility for excretion .
Mammalian Metabolism (Rats):
Environmental Persistence and Byproducts
Parameter | Bromoxynil Phenol | Bromoxynil Octanoate | Sources |
---|---|---|---|
Soil DT₅₀ (aerobic) | 10 days | 1–3 days | |
Water DT₅₀ | 30 days | 1–34 days | |
Bioaccumulation (logKow) | 2.8 | 5.4 |
科学的研究の応用
Chemistry
BROMOXYNILPHENOL is utilized as a reagent in organic synthesis. It plays a crucial role in the formation of complex molecules, facilitating various chemical reactions that are essential for developing new compounds.
Biology
The compound has been studied for its potential biological activities, particularly its antimicrobial and anticancer properties. Research indicates that this compound exhibits significant activity against certain pathogens, making it a candidate for further exploration in microbiology and pharmacology .
Medicine
In the medical field, this compound is investigated for its potential use as a precursor in drug development. Its structural characteristics allow it to be modified into active pharmaceutical ingredients, providing a pathway for new therapeutic agents.
Industry
The compound is also employed in the synthesis of specialty chemicals and materials. Its versatility makes it valuable in industrial applications where specific chemical properties are required.
Biochemical Properties
This compound impacts various biochemical processes:
- Cellular Effects : Studies show that this compound influences cellular processes and has effects on different cell types.
- Molecular Mechanism : The molecular interactions include binding with biomolecules and enzyme modulation, which can alter gene expression patterns.
- Dosage Effects : Research indicates that the effects of this compound vary significantly with dosage in animal models, suggesting a need for careful dosing in therapeutic applications .
Table 1: Applications of this compound
Application Area | Description |
---|---|
Chemistry | Reagent in organic synthesis |
Biology | Antimicrobial and anticancer research |
Medicine | Precursor for active pharmaceutical ingredients |
Industry | Synthesis of specialty chemicals |
Pathogen | Activity Level | Reference |
---|---|---|
Staphylococcus aureus | Significant | |
Methicillin-resistant Staphylococcus aureus (MRSA) | Moderate |
Case Studies
- Antibacterial Activity : A study explored the antibacterial properties of bromophenol derivatives, revealing that compounds similar to this compound demonstrated significant effectiveness against Staphylococcus aureus and MRSA. The therapeutic index was notably high, indicating low toxicity to human cells while effectively inhibiting bacterial growth .
- Drug Development Potential : Research has highlighted the potential of this compound as a precursor for novel drugs targeting resistant bacterial strains. This aligns with current trends in pharmaceutical research aimed at combating antibiotic resistance through innovative compounds .
作用機序
The mechanism of action of BROMOXYNILPHENOL depends on its specific application. In biological systems, it may interact with molecular targets such as enzymes or receptors, modulating their activity. The oxime group can form stable complexes with metal ions, which may be relevant in its mechanism of action.
類似化合物との比較
Similar Compounds
Ethyl alpha-(hydroxymethylene)-beta-oxohydrocinnamate: Lacks the ethoxy group.
Ethyl beta-oxohydrocinnamate: Lacks both the oxime and ethoxy groups.
Sodium alpha-(ethoxyhydroxymethylene)-beta-oxohydrocinnamate: Similar but without the oxime group.
Uniqueness
The presence of both the ethoxy and oxime groups in BROMOXYNILPHENOL makes it unique. This combination of functional groups can impart distinct chemical reactivity and biological activity, setting it apart from similar compounds.
生物活性
Bromoxynil phenol, a nitrile-group herbicide, is primarily known for its role in agricultural applications as a selective herbicide. This article delves into the biological activity of bromoxynil phenol, focusing on its toxicological profile, metabolic pathways, and potential health effects based on diverse research findings.
Bromoxynil phenol is characterized by its ability to inhibit photosynthesis and mitochondrial oxidative phosphorylation in plants. This mechanism leads to the disruption of energy production, ultimately resulting in plant death. The compound is used primarily against broadleaf weeds and is applied in various crops, including cotton and cereals .
Acute Toxicity
Bromoxynil phenol exhibits moderate acute toxicity. In laboratory studies, it has shown:
- Oral Toxicity : LD50 values range from 300 to 2000 mg/kg in rats, indicating moderate toxicity.
- Dermal Toxicity : Low dermal toxicity was observed in rabbits.
- Inhalation Toxicity : Moderate inhalation toxicity was noted .
Chronic Toxicity
Chronic exposure studies have revealed significant findings:
- Liver Effects : The liver is the primary target organ for bromoxynil toxicity. Long-term dietary administration in animal models has resulted in liver hyperplasia and other biochemical changes at doses as low as 1.5 mg/kg/day .
- Developmental Effects : In reproductive studies, bromoxynil has been linked to skeletal malformations (e.g., supernumerary ribs) and decreased pup weights without affecting overall fertility .
Metabolism and Pharmacokinetics
The metabolism of bromoxynil phenol involves hydrolysis and conjugation pathways. In rats, it is rapidly absorbed after oral administration, with metabolites such as bromoxynil sulfate and glucuronide being excreted primarily through urine. Tissue residues were found to be low, indicating efficient excretion mechanisms .
Case Study 1: Liver Toxicity in Dogs
A one-year chronic toxicity study in dogs revealed that bromoxynil caused elevated liver weights and biochemical changes at doses of 1.5 mg/kg/day. A no-observed-adverse-effect level (NOAEL) was established at 0.3 mg/kg/day for body weight gain reductions .
Case Study 2: Developmental Toxicity in Rats
In a three-generation reproductive study, exposure to bromoxynil resulted in decreased parental body weights and reduced pup numbers. However, no significant effects on fertility or gestation length were observed, with a NOAEL of 1.5 mg/kg/day established for reproductive effects .
Risk Assessment
The Environmental Protection Agency (EPA) has conducted extensive risk assessments for bromoxynil phenol:
- Cancer Risk : Classified as a possible human carcinogen based on liver tumor data from animal studies.
- Dietary Exposure : The aggregate dietary risk from food and water exposure is estimated to be low, with no population subgroup exceeding safety thresholds .
Summary of Findings
Parameter | Findings |
---|---|
Chemical Class | Nitrile-group herbicide |
Acute Oral Toxicity (LD50) | 300 - 2000 mg/kg |
Chronic NOAEL (Dogs) | 0.3 mg/kg/day |
Developmental NOAEL (Rats) | 5 mg/kg/day |
Primary Target Organ | Liver |
Carcinogenic Potential | Possible human carcinogen |
特性
IUPAC Name |
sodium;diethyl 2-[(oxidoamino)-phenylmethylidene]propanedioate | |
---|---|---|
Source | PubChem | |
URL | https://pubchem.ncbi.nlm.nih.gov | |
Description | Data deposited in or computed by PubChem | |
InChI |
InChI=1S/C14H16NO5.Na/c1-3-19-13(16)11(14(17)20-4-2)12(15-18)10-8-6-5-7-9-10;/h5-9,15H,3-4H2,1-2H3;/q-1;+1 | |
Source | PubChem | |
URL | https://pubchem.ncbi.nlm.nih.gov | |
Description | Data deposited in or computed by PubChem | |
InChI Key |
QCQUOMIBMARNCT-UHFFFAOYSA-N | |
Source | PubChem | |
URL | https://pubchem.ncbi.nlm.nih.gov | |
Description | Data deposited in or computed by PubChem | |
Canonical SMILES |
CCOC(=O)C(=C(C1=CC=CC=C1)N[O-])C(=O)OCC.[Na+] | |
Source | PubChem | |
URL | https://pubchem.ncbi.nlm.nih.gov | |
Description | Data deposited in or computed by PubChem | |
Molecular Formula |
C14H16NNaO5 | |
Source | PubChem | |
URL | https://pubchem.ncbi.nlm.nih.gov | |
Description | Data deposited in or computed by PubChem | |
DSSTOX Substance ID |
DTXSID10910904 | |
Record name | Sodium {[3-ethoxy-2-(ethoxycarbonyl)-3-oxo-1-phenylprop-1-en-1-yl]amino}oxidanide | |
Source | EPA DSSTox | |
URL | https://comptox.epa.gov/dashboard/DTXSID10910904 | |
Description | DSSTox provides a high quality public chemistry resource for supporting improved predictive toxicology. | |
Molecular Weight |
301.27 g/mol | |
Source | PubChem | |
URL | https://pubchem.ncbi.nlm.nih.gov | |
Description | Data deposited in or computed by PubChem | |
CAS No. |
1089-84-5 | |
Record name | Hydrocinnamic acid, alpha-(ethoxyhydroxymethylene)-beta-oxo-, ethyl ester, beta-oxime, sodium salt | |
Source | ChemIDplus | |
URL | https://pubchem.ncbi.nlm.nih.gov/substance/?source=chemidplus&sourceid=0001089845 | |
Description | ChemIDplus is a free, web search system that provides access to the structure and nomenclature authority files used for the identification of chemical substances cited in National Library of Medicine (NLM) databases, including the TOXNET system. | |
Record name | Sodium {[3-ethoxy-2-(ethoxycarbonyl)-3-oxo-1-phenylprop-1-en-1-yl]amino}oxidanide | |
Source | EPA DSSTox | |
URL | https://comptox.epa.gov/dashboard/DTXSID10910904 | |
Description | DSSTox provides a high quality public chemistry resource for supporting improved predictive toxicology. | |
試験管内研究製品の免責事項と情報
BenchChemで提示されるすべての記事および製品情報は、情報提供を目的としています。BenchChemで購入可能な製品は、生体外研究のために特別に設計されています。生体外研究は、ラテン語の "in glass" に由来し、生物体の外で行われる実験を指します。これらの製品は医薬品または薬として分類されておらず、FDAから任何の医療状態、病気、または疾患の予防、治療、または治癒のために承認されていません。これらの製品を人間または動物に体内に導入する形態は、法律により厳格に禁止されています。これらのガイドラインに従うことは、研究と実験において法的および倫理的な基準の遵守を確実にするために重要です。